VACLAV SMIL
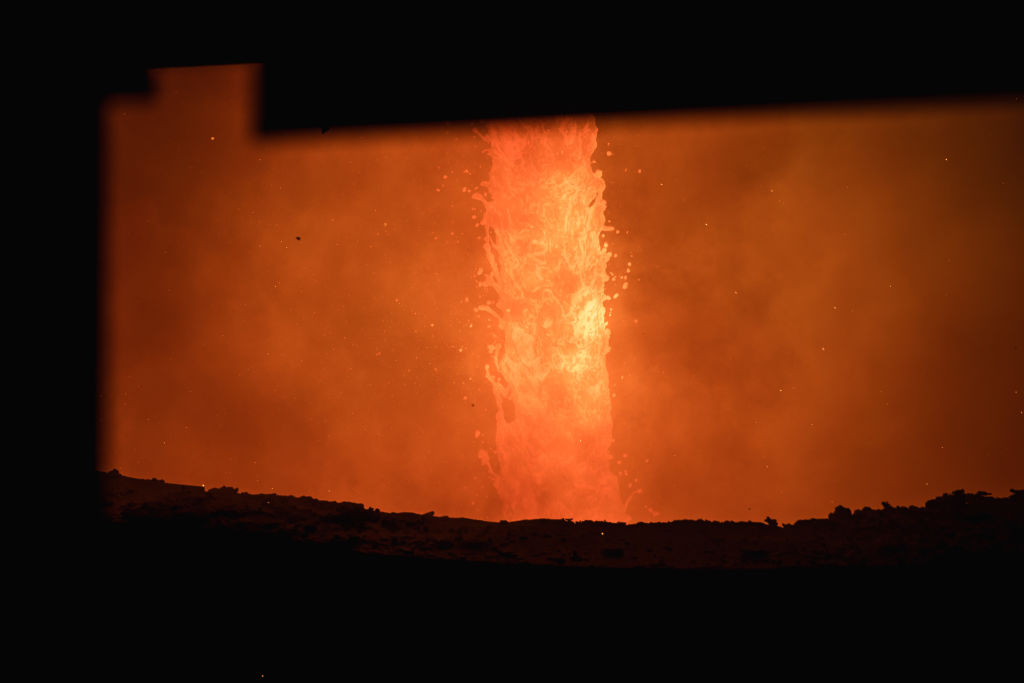
Modern societies would be impossible without mass-scale production of many man-made materials. We could have an affluent civilization that provides plenty of food, material comforts, and access to good education and health care without any microchips or personal computers: we had one until the 1970s, and we managed, until the 1990s, to expand economies, build requisite infrastructures and connect the world by jetliners without any smartphones and social media. But we could not enjoy our quality of life without the provision of many materials required to embody the myriad of our inventions.
Four materials rank highest on the scale of necessity, forming what I have called the four pillars of modern civilization: cement, steel, plastics, and ammonia are needed in larger quantities than are other essential inputs. The world now produces annually about 4.5 billion tons of cement, 1.8 billion tons of steel, nearly 400 million tons of plastics, and 180 million tons of ammonia. But it is ammonia that deserves the top position as our most important material: its synthesis is the basis of all nitrogen fertilizers, and without their applications it would be impossible to feed, at current levels, nearly half of today’s nearly 8 billion people.
The dependence is even higher in the world’s most populous country: feeding three out of five Chinese depends on the synthesis of this compound. This dependence easily justifies calling ammonia synthesis the most momentous technical advance in history: other inventions provide our comforts, convenience or wealth or prolong our lives—but without the synthesis of ammonia, we could not ensure the very survival of billions of people alive today and yet to be born.
Plastics are a large group of synthetic organic materials whose common quality is that they can be molded into desired shapes—and they are now everywhere. As I type this, the keys of my Dell laptop and a wireless mouse under my right palm are made of acrylonitrile butadiene styrene, I sit on a swivel chair upholstered in a polyester fabric, and its nylon wheels rest on a polycarbonate carpet protection mat that covers a polyester carpet. But plastics are now most indispensable in health care in general and in hospitals in particular. Life now begins (in maternity wards) and ends (in intensive care units) surrounded by plastic items made above all from different kinds of PVC: flexible tubes (for feeding patients, delivering oxygen, and monitoring blood pressure), catheters, intravenous containers, blood bags, sterile packaging, trays and basins, bedpans and bed rails, thermal blankets.
Steel’s strength, durability, and versatility determines the look of modern civilization and enables its most fundamental functions. This is the most widely used metal and it forms countless visible and invisible critical components of modern civilization, from skyscrapers to scalpels. Moreover, nearly all other metallic and non-metallic products we use have been extracted, processed, shaped, finished, and distributed with tools and machines made of steel, and no mode of today’s mass transportation could function without steel. The average car contains about 900 kilograms of steel and before Covid-19 struck the world was making nearly 100 million vehicles a year.
Cement is, of course, the key component of concrete: combined with sand, gravel and water it makes the most massively deployed material. Modern cities are embodiments of concrete, as are bridges, tunnels, roads, dams, runways and ports. China now produces more than half of the world’s cement and in recent years it makes in just two years as much of it as did the United States during the entire 20th century. Yet another astounding statistic is that the world now consumes in one year more cement than it did during the entire first half of the 20th century.
And these four materials, so unlike in their properties and qualities, share three common traits: they are not readily replaceable by other materials (certainly not in the near future or on a global scale); we will need much more of them in the future; and their mass-scale production depends heavily on the combustion of fossil fuels, making them major sources of greenhouse gas emissions. Organic fertilizers cannot replace synthetic ammonia: their low nitrogen content and their worldwide mass are not enough even if all manures and crop residues were recycled. No other materials offer such advantages for many lightweight yet durable uses as plastics. No other metal is as affordably strong as steel. No other mass-produced material is as suitable for building strong infrastructure as concrete (often reinforced with steel).
As for the future needs, high-income countries could reduce their fertilizer use (eating less meat, wasting less), and China and India, the two heavy users, could also reduce their excessive fertilizer applications, but Africa, the continent with the fastest-growing population, remains deprived of fertilizers even as it is already a substantial food importer. Any hope for its greater food self-sufficiency rests on the increased use of nitrogen: after all, the continent’s recent usage of ammonia has been less than a third of the European mean. More plastics will be needed for expanding medical (aging populations) and infrastructural (pipes) uses and in transportation (see the interior of airplanes and high-speed trains). As is the case with ammonia, steel consumption has to rise in all low-income countries with underdeveloped infrastructures and transportation. And much more cement will be needed to make concrete: affluent countries to fix decaying infrastructures (in the US all sectors where concrete dominates, including dams, roads, and aviation get a D grade in nationwide engineering assessments), in low-income countries to expand cities, sewers and transportation.
Moreover, the unfolding transition to renewable energies will demand huge amounts of steel, concrete and plastics. No structures are more obvious symbols of “green” electricity generation than large wind turbines—but their foundations are reinforced concrete, their towers, nacelles, and rotors are steel, and their massive blades are energy-intensive—and difficult to recycle—plastic resins, and all of these giant parts must be brought to the installation sites by outsized trucks (or ships) and erected by large steel cranes, and turbine gearboxes must be repeatedly lubricated with oil. These turbines would generate truly green electricity only if all of these materials were made without any fossil fuels.
Fossil fuels remain indispensable for producing all of these materials.
Ammonia synthesis uses natural gas both as the source of hydrogen and as the source of energy needed to provide high temperature and pressure. Some 85% of all plastics are based on simple molecules derived from natural gas and crude oil, and hydrocarbons also supply energy for syntheses. Production of primary steel starts with smelting iron ore in blast furnace in the presence of coke made from coal and with the addition of natural gas, and the resulting cast iron is made into steel in large basic oxygen furnaces. And cement is produced by heating ground limestone and clay, shale in large kilns, long inclined metal cylinders, heated with such low-quality fossil fuels as coal dust, petroleum coke and heavy fuel oil.
As a result, global production of these four indispensable materials claims about 17 percent of the world’s annual total energy supply, and it generates about 25 percent of all CO2 emissions originating in the combustion of fossil fuels. The pervasiveness of this dependence and its magnitude make the decarbonization of the four material pillars of modern civilization uncommonly challenging: replacing fossil fuels in their production will be far more difficult and costly than generating more electricity from renewable (mainly wind and solar) conversions. Eventually, new processes will take over— but currently there are no alternatives that could be deployed immediately to displace large shares of existing global capacities: their development will take time.
Synthesis of ammonia and smelting of steel could both be based on hydrogen rather than on natural gas and coke. We know how to do that—but it will take some time before we could produce hundreds of million tons of green hydrogen derived from the electrolysis of water by using wind or solar electricity (virtually all of today’s hydrogen is derived from natural gas and coal). The best forecast is that green hydrogen would supply 2% of the world’s energy consumption by 2030, far below the hundreds of million tons that will eventually be needed to decarbonize ammonia and steel production. In contrast, decarbonization of cement production can only go so far by using waste materials and biomass, and new processes have to developed and be commercialized to make cement CO2-free. Similarly, there is no simple way to decarbonize plastic production, and the measures will range from plant feedstocks to more recycling and to substitutions by other materials.
And beyond these four material pillars new and highly energy-intensive material dependencies are emerging and electric cars are their best example A typical lithium car battery weighing about 450 kilograms contains about 11 kilograms of lithium, nearly 14 kilograms of cobalt, 27 kilograms of nickel, more than 40 kilograms of copper, and 50 kilograms of graphite—as well as about 181 kilograms of steel, aluminum, and plastics. Supplying these materials for a single vehicle requires processing about 40 tons of ores, and given the low concentration of many elements in their ores it necessitates extracting and processing about 225 tons of raw materials. And aggressive electrification of road transport would soon require multiplying these needs by tens of millions of units per year!
Modern economies will always be tied to massive material flows, whether those of ammonia-based fertilizers to feed the still-growing global population; plastics, steel, and cement needed for new tools, machines, structures, and infrastructures; or new inputs required to produce solar cells, wind turbines, electric cars, and storage batteries. And until all energies used to extract and process these materials come from renewable conversions, modern civilization will remain fundamentally dependent on the fossil fuels used in the production of these indispensable materials. No artificial intelligence designs, no apps, no claims of coming “dematerialization” will change that.
No comments:
Post a Comment