Ian Clay and Robert D. Atkinson
INTRODUCTION
The last decade was marked by dramatic evolution in China’s innovation capabilities and strategies, much of which was driven by the transition of Chinese Communist Party (CCP) and state leadership from Hu Jintao and Xi Jinping and the introduction of China’s latest major innovation policy framework: Made in China 2025 (MIC). This report updates an earlier ITIF report, applying more recent data to assess the progress China made during the previous decade with respect to the United States across a series of innovation indicators.1
Innovation means different things to different people, in part because there are so many different kinds of innovation. One kind is catch-up or copying innovation, wherein China has performed superbly. Another is new-to-the-world or frontier innovation. China’s capacity for the latter is one of the most important unknowns in the global economy. Many countries have tried and failed to make the transition from “imitator” to “innovator,” and China’s ability or inability to fully make that transition will largely define global geopolitical development in the decades to come. If China can surpass the United States in innovation—both catch-up and frontier—the global value chain (GVC) for the highest-value-added products stands to undergo a tremendous change. This would represent a serious economic and geopolitical challenge to the United States and its allies, particularly because of China’s predatory trade and innovation policy practices.
The Goal of This Report
This report looks back on the previous decade and gauges the progress China made relative to the United States in a series of innovation indicators. The indicators are grouped into three categories: innovation inputs, innovation outputs, and innovation outcomes. By reviewing a range of indicators, one can develop a better understanding of where China is or is not making progress, specifically relative to the United States.
Furthermore, many analyses of innovation focus on a collection of indicators that is too narrow. Specifically, many analyses devote too much attention to traditional measures of innovation such as research and development (R&D) intensity and patent output. While the accumulation of knowledge and inventiveness are certainly necessary for innovation, commercialization in the marketplace is an equally consequential part of the innovation process. Innovation is not just about who invents a technology but who can use it to deliver the best products or services to potential users. It is with this in mind that this report’s analysis of innovation indicators expands beyond just reviewing traditional innovation inputs and outputs and attempts to also gauge the outcomes that these inputs and outputs bring about in markets and society.
This report is structured as follows: The section on “China’s Innovation Policy History” describes the goals of China’s major innovation policy developments and the methods to achieve them. This is followed by a section on “China’s Ability to Innovate,” including a summary of the arguments for why China is not capable of innovation at the frontier—at least in the way developed economies are—and why these arguments hold less and less water by the year. Two indices are constructed—one considering indicators that account for the size of each economy and one only considering indicators that do not—and China’s scores relative to the United States’ at the beginning and end of the decade are reported to provide an overall measure of China’s progress. The individual innovation indicators and China’s performance in them relative to the United States over the previous decade follow, accompanied by brief analyses. Lastly, the general results, the message they convey, and what can be expected in terms of future developments are discussed, followed by a brief summary and conclusion of the report.
Why This Matters
The consequences of losing the competitive edge in advanced, high-value-added industries are different than those of losing that edge in low-skill industries for three primary reasons: barriers to re-entry, loss of good-paying jobs, and national security risks.
If its unitary cost of labor (the ratio of wages to productivity) were to fall enough, the United States could re-enter low-skill industries quite easily. Relatively little know-how and machinery are required to start producing in these industries, so market entrants could simply purchase the equipment and hire the labor necessary with few obstacles. However, this is not the case in advanced industries such as semiconductor or aerospace manufacturing. Entry into these industries requires high-skill labor, massive investments in specialized equipment, and, in many cases, the ability to tread water until enough know-how is acquired to take advantage of economies of scale. Take semiconductor manufacturing as an example. The process of manufacturing one dynamic random access memory (DRAM) chip consists of over 1,000 steps. Entering the DRAM market and gaining market share requires the procurement of specialized, complex machinery capable of carrying out these tasks, a tacit understanding of the manufacturing process by the firm’s workers (from the factory floor to the research laboratory), and the right innovation ecosystem (universities to train talent, a sufficient network of suppliers, etc.) to foster the industry. The firm may have to operate at a loss for some time until it has acquired enough know-how and become productive enough to capture the advantages of economies of scale required to become competitive in the international market. Thus, re-entering advanced, technology-intensive industries is far more difficult than re-entering lower-skill industries.
Beyond extensive barriers to re-entry, the loss of market share in advanced, high-value-added industries means a loss of jobs in these industries, which are typically much higher paying than those in other sectors. For example, the average salary of a worker in information technology (IT) sectors in the United States is approximately 75 percent higher than the average U.S. salary in general.2 Therefore, lost jobs in these industries mean not only temporary unemployment for affected workers but a long-term overall decrease in aggregate well-being.
Lastly, remaining competitive in advanced, technology-intensive industries is crucial for national security. The superiority of the U.S. military rests largely on its technological superiority. This extends beyond technology for the physical battlefield and now crucially includes areas such as cybersecurity and intelligence gathering. A loss of competitiveness in the production of technologies crucial to national defense and an increase in dependence on other nations to produce them means two things. First—and obviously—it means the United States becomes more dependent on other countries to supply its military. While this may be less concerning (though not unconcerning) if the production is shifted to an ally country, this dynamic would be incredibly concerning if the production were shifted to a country such as China, which, if not an outright adversary, is a at least a geopolitical rival. Second, even if the United States could reliably count on other countries to provide it with military technologies and supplies, its superiority would be diminished by definition. If the United States must rely on others for the development and production of defense technologies, then its military can only be as technologically advanced as its suppliers’. Again, this may be less concerning if those developing and producing the technologies are allies, but it would be unsettling if the developer were a country such as China.
Related to the issue of national security is that of economic security. The globalization of supply chains has yielded amazing benefits in efficiency and cost reduction as economies specialize in the activities in which they have a comparative advantage. However, as supply chain disruptions triggered by the COVID-19 pandemic have shown, globalization introduces economic fragility. This was especially evident for semiconductors, the shortage of which drove up prices in everything from automobiles to home appliances. The effect of the semiconductor shortage was so widespread because of its prevalence as an intermediate good and the lack of relatively close substitutes. Per a recent blog by the Federal Reserve Bank of St. Louis, one-quarter of U.S. manufacturing industries, accounting for 39 percent of total U.S. manufacturing output, use semiconductors as a direct input.3 It is therefore important to a country’s economic security to be active in these strategically important industries by remaining or becoming a competitively efficient producer (rather than through processes such as import substitution that will jeopardize efficiency and innovativeness).
General Results
Overall, China made notable progress relative to the United States. This progress was strongest and most widespread in innovation outputs and—unsurprisingly—in indicators not accounting for size, where it surpassed the United States. However, China made progress relative to the United States in all three innovation types, both when accounting for size and when not. In summary, China is beginning to make use of its massive economic and demographic endowments to eclipse the United States in gross output of innovation indicators (e.g., number of science and engineering articles published, number of doctoral degrees awarded, advanced-industry output, etc.). This has translated into slower but still significant progress in indicators accounting for the size of each country’s economy or population, where China still lags behind the United States.
CHINA’S INNOVATION POLICY HISTORY
2006–2010: Indigenous Innovation, Thousand Talents, and Strategic Emerging Industries
China’s modern innovation policies started in earnest with the “indigenous innovation” movement in the Medium- to Long-Term Program (MLP) for the Development of Science and Technology released in 2006. MLP defined indigenous innovation as “enhancing original innovation through co-innovation and re-innovation based on the assimilation of imported technologies.”4 Specifically, MLP and indigenous innovation constituted a strategy to address six key issues:
1. China’s weak capacity for commercial innovation
2. Insufficient technological capabilities in strategic economic and public areas such as resource utilization and public health
3. Overreliance on foreign technology in areas of financial, civil, and national security
4. The exodus of China’s top science and engineering talents
5. “Expropriation” by foreign firms in the form of royalties and licensing fees charged to Chinese producers
6. An increasing realization that appropriation of foreign technologies would not lead to sustained long-term economic growth5
To address these issues and to make China more technologically independent and innovative, MLP cited key economic sectors, technologies, and megaprojects that would receive the focus of China’s government. The sectors cited were energy, water, and mineral resources; the environment; agriculture; manufacturing; transportation; information and services; population and health; urbanization; and public and national security. The technologies cited were biotechnology, IT, advanced materials, advanced manufacturing, advanced energy technology, marine technology, laser technology, and space technology. And the megaprojects to be funded by the state focused on protein science, nanotechnology, quantum physics, and developmental and reproductive science.6
MLP also laid out explicit goals to be achieved by 2020. The Chinese government sought for the nation’s R&D intensity (R&D expenditures as a share of gross domestic product [GDP]) to reach 2.5 percent and for basic research to comprise 15 percent of such expenditures. Additionally, the government sought to become first in the world with respect to patents filed and academic articles published.7
To achieve these goals, China’s government implemented and promoted a range of protectionist and filching policies, most of which fly in the face of the World Trade Organization’s (WTO’s) rules. The first of these policies was an expansion of the now-infamous forced technology transfers and intellectual property (IP) theft by Chinese companies. Among the primary measures undertaken to achieve MLP’s goals was “[adjusting and improving] national policies on industrial technology so as to reinforce the assimilation and absorption of imported technologies and re-innovation.”8 The second of these policies directed the raising of implicit trade barriers such as stricter quality and assurance testing and industrial and technology standards for foreign companies than those faced by domestic firms. The third policy embraced enhanced subsidies to state-owned enterprises (SOEs), specifically those in the sectors producing the technologies listed above. The final major policy used to achieve MLP’s goals was the introduction of a “Buy China” requirement for government procurement of the following technologies: computers and applications equipment, communications products, modern office equipment, software, new energy and related devices, and high-efficiency and energy-saving products. This “Buy China” provision required that all procured goods and services in these technologies be produced using Chinese-owned IP and a commercial trademark registered in China.9
Three of China’s policies—forced technology transfers and IP theft, implicit barriers on would-be imports, and subsidies to exporting SOEs—are explicitly against WTO laws. Since the Chinese government had not (and still has not) signed WTO’s Agreement on Government Procurement (GPA), this final policy is not technically in conflict with China’s WTO commitments. That said, China’s representative in WTO-accession negotiations made clear that the country intended to become a GPA member soon after attaining WTO membership and would submit an offer to do so “as soon as possible” upon accession.10 So here, too, China flouted its WTO promises.
As such, multinational corporations seeking to expand their business in China voiced their opposition to MLP, and “indigenous innovation” was seen as a thinly disguised pretense to introduce mercantilist policies. Affected parties took particular issue with the inclusion of the terms “co-innovation” and “re-innovation” in the government’s definition of indigenous innovation, fretting over the technical and implied definitions and (often correctly) fearing that they referred to forced transfers of technologies and trade secrets in return for access to the Chinese market. Additionally, Chinese-national scientists and engineers abroad expressed concern about the government’s planned megaprojects, arguing that such massive, state-run undertakings would diminish competition among involved scientists, increase bureaucratic inefficiencies, and bias results toward the preferences of China’s Ministry of Science and Technology.11
Two years following the release of MLP, the Chinese government announced its Thousand Talents Program to address China’s inability to retain and attract science and engineering talent. The program was launched first to attract top ex-patriot professors and scientists in the West to return to China and, starting in 2010, to attract foreign nationals as well. The benefits offered as part of the program include a starting bonus of over $150,000 and the ability to apply for a $450,000 to $800,000 research grant. Foreign nationals accepted as part of the program receive additional benefits such as housing subsidies, paid-for trips home, and a job or stipend for their spouse. 12 As of 2018, the program had attracted over 7,000 professors and scientists.
The Decision on Accelerating the Cultivation and Development of Strategic Emerging Industries—or, more simply, the Strategic Emerging Industries (SEI) strategy—updated MLP by announcing seven key sectors in which China hoped to become a world leader: energy efficiency and environmental technology, next-generation IT, biotechnology, high-end equipment manufacturing, new energy, new materials, and new-energy vehicles. Funding and administrative support for these technologies were primarily shouldered by the country’s local and provincial governments rather than the central government in Beijing.
2015: Made in China 2025
The next and most-recent major milestone in China’s innovation policy timeline was the announcement of MIC. Rather than a simple extension or update of MLP or SEI, MIC shifts the focus of innovation policy toward putatively market outcomes and enhancing the entire manufacturing process, places more emphasis on measurable goals, and carves out a greater role for market mechanisms (at least for Chinese firms).13 The broad goals laid out in MIC are to introduce innovation-driven “smart” manufacturing through the implementation of industrial robots and advanced IT (specifically the Internet of Things); to attract and nurture human capital; to gain market share in high-value-added parts of the GVC; to strengthen IP rights and protections for small and medium-sized enterprises (SMEs) and to make more strategic use of IP; the harmonization of Chinese companies’ technology standards with those of the international community to increase exports; and increased international brand recognition of national champion firms.14 As Scott Kennedy of the Center for Strategic and International Studies (CSIS) points out, MIC is more like Germany’s “Industry 4.0” than it is MLP, at least in its intent to modernize manufacturing.15
Figure 1: Semi-official targets for domestic market share of Chinese products under Made in China 202516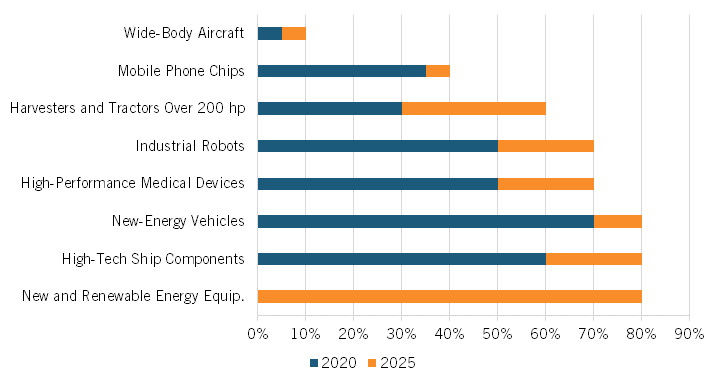
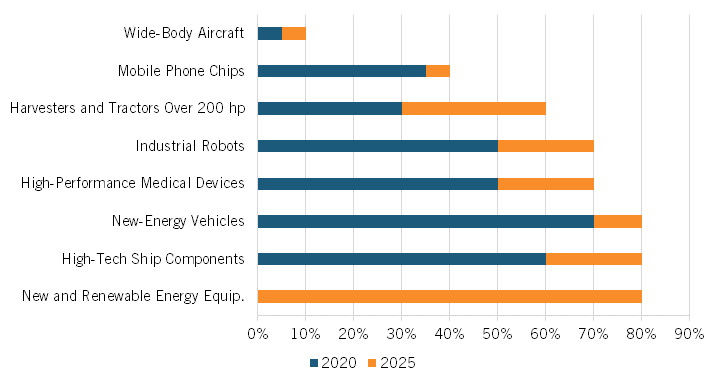
Like MLP, MIC enumerates specific priority sectors. It also introduced benchmarks to hit in these industries by 2020 or 2025. The priority sectors mentioned are new advanced IT; automated machine tools and robotics; aerospace and aeronautical equipment; maritime equipment and high-tech shipping; modern rail transport and related equipment; new-energy vehicles and related equipment; power equipment; agricultural equipment; new materials; and biopharmaceuticals and advanced medical products.17 The specific goals in MIC are (or were) that the domestic content of core components and materials reach 40 percent by 2020 and 70 percent by 2025; to establish 15 innovation centers by 2020 and 40 by 2025; for corporate R&D intensity to reach 1.68 percent by 2025; for labor productivity to increase by 7.5 percent per year between 2015 and 2020; and for energy and water consumption per unit of value added to decrease by 35 percent by 2025.18
MIC represents an insightful shift in focus for China’s innovation policy. Innovation is about much more than just the number of academic publications or patents a society produces (although these are important), especially if such activity is more of a response to government incentives than to market incentives. Rather, the oft-forgotten aspect of the innovation process is bringing the invention to market, or the implementation, both in general and, importantly for China, at scale. Thus, this shift in focus toward market outcomes and commercialization rather than pure invention indicates China’s ability to see the whole picture.
China’s innovation policies are centered not necessarily on increasing productivity and technical know-how to move up the value chain, but to supplant foreign competitors and substitute imports in the industries it deems necessary.
Many outsiders fear that MIC reiterates China’s commitment to protectionism to achieve its economic goals. While MIC places more emphasis on market mechanisms by strengthening IP protections for SMEs and liberalizing firms’ setting of technology standards, the enhanced IP protections appear to only serve domestic enterprises, and the liberalized technology standards were implemented to increase domestic firms’ exports. Moreover, the government’s explicit desire to establish national champion firms and support SOEs in internationally important sectors indicates both that the government will still very much play a commanding role in the Chinese economy and the extent to which multinational firms are allowed to participate in it.
China is well within its right to develop and implement a strategy to boost its competitiveness and innovativeness, and ITIF would advise all nations to do so.19 However, China appears determined to subsidize its national champions and restrict market access to foreign competitors in advanced industries where it cannot achieve a comparative advantage. First and foremost, China’s innovation policies are centered not necessarily on increasing productivity and technical know-how to move up the value chain, but to supplant foreign competitors and substitute imports in the industries it deems necessary. This fundamental goal is encapsulated in MIC, prompting the United States Trade Representative to describe the strategy as follows:
While ostensibly intended simply to raise industrial productivity through more advanced and flexible manufacturing techniques, Made in China 2025 is emblematic of China’s evolving and increasingly sophisticated approach to “indigenous innovation,” which is evident in numerous supporting and related industrial plans. Their common, overriding aim is to replace foreign technologies, products, and services with Chinese technologies, products, and services in the China market through any means necessary to enable Chinese companies to dominate international markets [emphasis added].20
CHINA’S ABILITY TO INNOVATE
A Common Misconception: China Cannot Innovate
There exists a widespread view that China is incapable of “true” innovation, at least at the frontier. In general, the primary reasons given in support of this view are an education system that encourages rote memorization and represses creative expression, a risk-averse culture centered around a reverence for authority that is not conducive to disruption or drastic change, weak IP protections, and inefficient state involvement in markets. Proponents of these arguments believe that while China’s economic rise is impressive, it is bound to be a copier of innovations from the West, at least for the foreseeable future.
Examples of such arguments being used to dismiss the potential of China’s innovation capacity abound. In a 2014 article for The Diplomat, Kings College London Professor of Chinese Studies Kerry Brown wrote:
The Chinese government under Xi can pour all the money they want into vast research and development parks, churning out any number of world class engineers and computer programmers. Even with all of this effort, however, China is likely to produce few world class innovative companies. The fundamental structural problem is that the role of the state and government in China is still very strong…. The system that China currently has still rewards conformity.21
Former Hewlett Packard CEO Carly Fiorina claimed, “Although the Chinese are a gifted people, innovation and entrepreneurship are not their strong suits. Their society, as well as their education system, is too homogenized and controlled to encourage imagination and risk-taking.”22 TechNode editor Jason Lim wrote, “Most Chinese start-ups are not founded by designers or artists, but by engineers who don’t have the creativity to think of new ideas or designs.”23 And only four years ago, Michael Pettis, a professor at the Guanghua School of Management at Peking University, bluntly stated, “This is not a country we can expect major innovations from. In the west we don’t have enough confidence about this. How many governments in the world have decided they’re going to become major innovation centers? None of them have succeeded.”24
Scott Kennedy of CSIS is also skeptical of China’s ability to turn willpower and resource allocation into innovation. Citing the lack of growth in China’s output score relative to its input score in the World Intellectual Property Organization’s Global Innovation Index (GII) between 2009 and 2016, Kennedy dubbed China a “fat tech dragon,” since its apparent inability to turn inputs into outputs is analogous to a low metabolism.25 Kennedy also points out that, while plentiful, Chinese patents are of relatively little practical use. Licensing revenues from the use of patents are still minuscule, and the surge in patents filed is a response to government rather than market incentives. Despite these issues, Kennedy acknowledges that China now graduates more scientists and engineers from its universities than does any other country (more than 1.8 million undergraduates in 2018), a higher percentage of bank loans are going to private businesses rather than SOEs, and IP protections are steadily expanding.26 Nevertheless, Kennedy argues, innovation appears to be a secondary goal to market expansion overseen by the state.
The Reality: China Can Innovate
Are these arguments correct? In short, no—but as with most points of debate, the truth is more nuanced. To understand why these arguments are at best incomplete, it is helpful to first talk about what innovation is. Innovation is often used interchangeably with invention, specifically ground-breaking invention. This is evident both from the quotes previously provided and from the importance placed on R&D intensity and patent output as traditional measures of innovation. However, this view of innovation misses the forest for the trees. While the development of novel products and processes is obviously an important aspect of innovation, effective commercialization is at least as important.
A helpful example is that of the smartphone. Nokia invented the first touchscreen smartphone, the Nokia 7710, a full two years before Apple released the iPhone, and it was the first to offer a connected gaming-oriented app store, which Apple used as its prototype for the App Store. According to those arguments, of the two companies in this anecdote, only Nokia was actually innovative. Apple was simply a copier, not a creator. However, this view of innovation ignores the vital aspects that made Apple and its iPhone more successful than its competitor. Namely, Apple offered a better product by providing an ecosystem that integrated the iPhone with other Apple products such as iTunes and the App Store (or, more specifically, the operating system on which third-party developers built their apps).27 Apple’s marketing and knowledge of its customers’ preferences also helped Apple turn the iPhone and subsequent products into fashion and status symbols. Now iPhones make up 62 percent of the premium smartphone market (defined as devices costing at least $400) while the Nokia 7710 exists only as a collectible.28
In effect, Apple leveraged an innovative technology to create superior customer experiences it then monetized through a superior business model to supplant the technology’s original creator. It could do so because innovation is not just about who is first; it is more importantly about who can deliver a superior product (a function of the actual good or service, the customer experience, the price, etc.), which manifests itself in market outcomes.
While the development of novel products and processes is obviously an important aspect of innovation, effective commercialization is at least as important.
A better understanding of innovation is provided by the McKinsey Global Institute’s (MGI’s) The China Effect on Global Innovation, which separates innovations into four categories: efficiency-driven, customer-focused, engineering-based, and science-based.29 The report concludes that with its proven ability to produce goods at scale, adapt products to the Chinese market, and develop and adopt digital infrastructures such as mobile payment systems and e-commerce (sometimes even before more-developed economies), China has demonstrated that it is capable of efficiency-driven and customer-focused innovations. The challenge before it, the authors argue, is to catch up in the areas of engineering- and science-based innovations.
However, recent developments indicate China is doing just that. For example, China became the world’s largest automobile producer in 2009, thanks to government support after designating it a priority industry, and is now also the world’s largest producer and exporter of electric vehicles.30 China landed the first probe on the dark side of the moon in 2019, it began launching the components of its Tiangong space station in 2021, and current NASA Administrator Bill Nelson recently stated that China may land astronauts on the moon before the United States is itself able to return.31 China has recently added to the pantheon of tech giants by producing companies such as Alibaba, Baidu, Huawei, and Tencent, and eight of the ten fastest companies to reach a $1 billion valuation are Chinese.32 As recently as 2017, China possessed the most powerful supercomputer in the world, and it currently boasts two of the top 10 most powerful, although the true extent of China’s supercomputing capabilities is now unknown.33
An additional example of Chinese frontier innovativeness, notably in an area in which China transitioned from a copier to a leader, is high-speed rail (HSR). China first developed its HSR network with the help of imports and technology transfers from European and Japanese companies. It opened its first railway—between Beijing and Tianjin—in the summer of 2008 in time to show it off to the world as it hosted that year’s Summer Olympics. Since then, China’s HSR network has ballooned and is by far the largest in the world with over 23,500 miles of rail.34 It has also developed the world’s fastest autonomous train, capable of speeds up to 217 miles per hour, and one of China’s rail-producing SOEs, CRRC, has become the world’s largest producer of railway vehicles and related technologies. China has recently developed a prototype for a “mag-lev” train—a train suspended above its tracks via a magnetic field—that it claims is capable of speeds up to 385 miles per hour.35 In the coming years, China’s HSR network will expand both to its western regions and its regional neighbors’ lands to further integrate markets and ingrain the country’s less-developed western half into its supply network.
China became the world’s largest automobile producer in 2009 and is now also the world’s largest producer and exporter of electric vehicles.
Beyond just these specific examples, China’s progress is demonstrating itself through broader measures of innovation, such as the aforementioned GII. While Kennedy dubbed China the “fat tech dragon” because of the alleged imbalance in growth between its innovation input score and its innovation output score, this analysis neglects the fact that in the 2016 edition of the GII, China’s output score was higher than at any other point between 2009 and 2016 (the years Kennedy considers). Furthermore, China’s “efficiency” score, defined as the ratio of a nation’s output score to its input score (or, put another way, a nation’s innovation metabolism), ranked seventh among all nations.36 For reference, Switzerland, which ranked first overall, had the 5th highest efficiency score, Germany had the 9th highest, the United States had the 25th highest, and Japan had the 65th highest.
In the recently released 2022 edition of the GII, China ranks 11th overall (and Hong Kong ranks 14th overall), ahead of countries such as Japan (13th) and Canada (15th).37 Moreover, China ranks just 21st in input score and 8th in output score, and its output score was well above the 2016 level. China’s GII-defined strengths are “Trade, diversification, & market share” (an innovation input for which China ranks third) and “Intangible assets” (an innovation output for which China ranks second).
However, these high marks deserve a qualification. China is bound to score well in “Trade, diversification, & market share” since one of the three indicators in that category is purchasing power parity (PPP)-adjusted GDP (China has the largest)—though it also ranks second in domestic industry diversification. Additionally, China’s score in “Intangible assets” is largely propped up by its trademark output. Kennedy’s point remains valid (at least for now) that China’s IP is currently of little value and patent and trademark output is largely a response to government rather than market incentives. This is further evidenced by the fact that China ranks only 35th in IP receipts as a percentage of total trade (whereas the United States ranks 4th). Nevertheless, China still scores remarkably well in market-outcome indicators, ranking 4th in high-tech exports as a percentage of total trade and, contrary to arguments about its lack of creativeness, notching the top spot in creative goods exports as a percentage of total trade. China scores 14th in “Creative outputs” as a general category.38
This is not to say that China is now among the most fundamentally innovative economies in the world or that there are no further obstacles in its way to getting there. Its R&D expenditures and patent outputs are still largely a reflection of the government’s objectives rather than the economy’s inventive capabilities (more on this later). Although financing has expanded to private as well as SOEs, and IP protections are being strengthened (at least for domestic enterprises), state involvement in the Chinese economy will almost certainly hinder productivity growth, and a trade-off between scale and efficiency in priority sectors will likely persist. This may be further exacerbated by Xi Jinping’s ongoing crusade to consolidate the state’s power and strengthen its influence in both economic and social matters. This crusade will now face fewer internal obstacles after Xi’s consolidation of power at the recent 20th National Congress of the CCP.
But it is naรฏve to ignore recent developments simply because they run contrary to preconceived notions of Chinese culture and capabilities. A nation that competes with—and in some cases beats—world leaders in areas such as advanced computing technology, space exploration, and e-commerce despite having a real GDP per capita roughly 30 percent less than that of the United States in 1947 cannot be written off.39 Rather, China’s progress toward frontier innovation and whatever challenges that poses to the United States and its allies must be carefully and seriously considered. To that end, China’s progress relative to the United States across a series of innovation indicators that attempt to capture the entirety of innovation (inputs, outputs, and outcomes) during the previous decade is measured and discussed below.
INNOVATION INDICATORS
The indicators considered are sorted into three categories. The first is innovation inputs, which consist of the resources and the quality of the institutions designed to bring about increases in an economy’s stock of knowledge. The second is innovation outputs, or the discovered knowledge the inputs produced. The third is innovation outcomes, which are the effects from the implementation of the discovered knowledge that manifest themselves in production and in adoption of new technologies.
OVERALL SCORES
ITIF created two indices to quantify China’s overall progress across the indicators considered: one measuring China’s progress relative to the United States on a proportional basis (accounting for the size of its economy, population, etc.) and another measuring China’s progress relative to the United States in terms of gross output. In cases where there was significant overlap among the indicators in a category (e.g., the number of undergraduate degrees awarded overall and in specific fields of study), only the indicator deemed to be most important was included in composite index calculations (e.g., science and engineering degrees were included instead of the total number of degrees). For both the proportional and gross output indices, scores were computed for each of the indicator types (inputs, outputs, and outcomes), and the overall index score was computed by giving inputs and outputs a weight of 25 percent each and outcomes a weight of 50 percent. The appendix contains a full list of the indicators used and their respective weights.
Figure 2 shows China’s progress in proportional indicators. The contributions of the indicator types are calculated as the weighted average of China’s scores relative to the United States across the considered indicators multiplied by that indicator type’s weight. China’s score in this index was 57.6 percent that of the United States in 2010. By 2020, this increased by almost one-third to 75 percent, thanks mostly to progress in innovation outputs. In other words, on a proportional basis, China is now roughly 75 percent as advanced in innovation and advanced-industry production as the United States. If this relative growth continues apace, China will surpass the United States by 2035.
Figure 2: China’s indicator index score relative to the United States on a proportional basis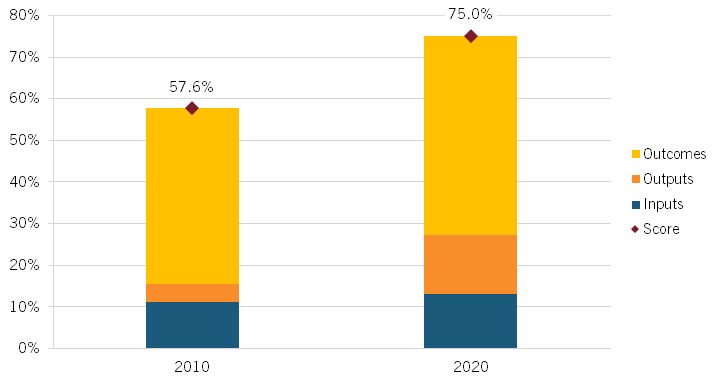
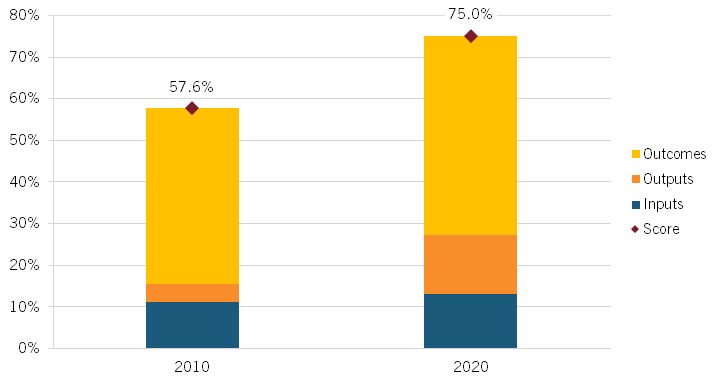
The contribution of the output indicators to China’s proportional index score more than tripled over the decade, thanks to rapid increases in China’s output of science and engineering articles and patents, the influence of China’s research, and China’s international IP receipts relative to GDP. This is especially notable when considering China’s score in outputs relative to the United States is only given a weight of 25 percent. While China also made progress in proportional innovation inputs and outcomes, this contributed much less to the increase in China’s score relative to the United States.
Similarly, figure 3 shows China’s relative progress in gross-output indicators. As these indicators do not account for China’s rapid growth in GDP in the last decade, China’s progress relative to the United States here is more pronounced. In 2010, China’s score in this index was 77.8 percent that of the United States. By 2020, this had grown by approximately four-fifths to 139.2 percent. In other words, China’s gross innovation capabilities (e.g., R&D expenditures, venture capital (VC) investments, advanced-industry output, patent output, etc.) are now almost 40 percent greater than those of the United States.
Figure 3: China’s indicator index score relative to the United States in gross output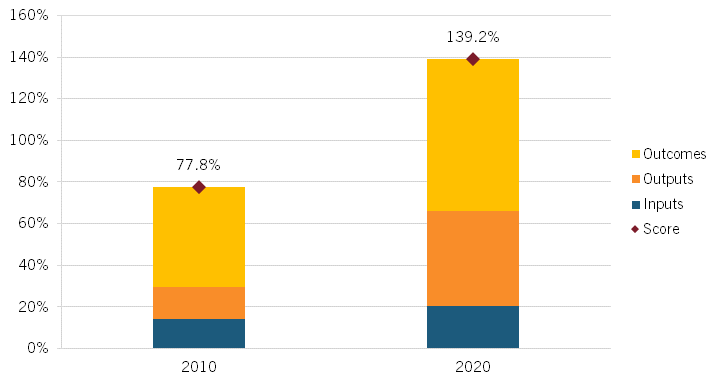
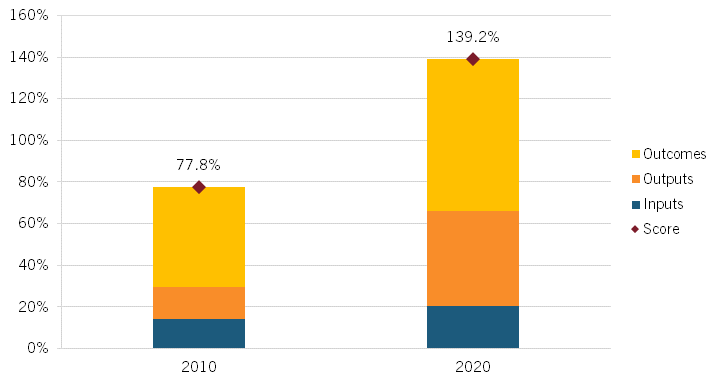
As with the proportional index, innovation outputs are the biggest contributors to this increase—again, with the innovation type’s contribution almost tripling—and now innovation outcomes contribute significantly as well (though it should again be noted that innovation outcomes’ weight is twice that of innovation inputs’ and innovation outputs’). The growth in the contribution of outputs to China’s score relative to the United States in the gross-output index is very similar to that in the proportional index, since the proportional innovation outputs account for population and China’s population was virtually unchanged relative to the United States’ during the past decade (with China maintaining approximately 4.3 times the population of the United States). Innovation outcomes contribute much more in this index, since the growth in China’s value added and exports in advanced industries relative to the United States’ is not mitigated by China’s rapid overall economic growth over the last decade. Again, while China made progress in gross innovation inputs relative to the United States, that progress was much more muted than that made in gross outputs and outcomes.
Though the two indices measure different (but highly related) things, they both convey that China’s biggest progress relative to the United States was in the category of innovation outputs. With respect to innovation inputs and innovation outcomes, this depends on which group of indicators one considers. With respect to proportional indicators, China made slightly more progress in innovation inputs than outcomes, but with respect to gross-output indicators, China made much more progress in innovation outcomes.
INNOVATION INPUTS
R&D
R&D is necessary to discover and adapt the knowledge necessary for future innovations and to create new products and processes.
R&D Expenditures
Figure 4 shows China’s R&D expenditures relative to the United States’ along with the relative expenditures of R&D’s components: basic research, applied research, and experimental development. Between 2010 and 2020, China’s R&D expenditures increased from $104.3 billion to $352.9 billion, and from 25 percent to 49 percent of U.S. levels, respectively. China’s growth relative to the United States’ was faster in the first half of the decade than in the second as growth in China’s R&D expenditures slowed from 16.9 percent per year in 2010–2015 to 9.2 percent per year in 2015–2020; the United States’ increased from 4.4 percent per year to 7.3 percent per year.
Basic research involves the discovery of foundational scientific knowledge upon which to build technologies. Unlike with total R&D expenditures, China’s basic research expenditures relative to the United States’ increased steadily throughout the decade, from 6.4 percent to 19.7 percent of the U.S. level ($4.8 billion to $21.2 billion) between 2010 and 2020. Although it made steady progress, basic research made up only 6 percent of China’s total R&D expenditures in 2020, well below its MLP goal of 15 percent.41
Applied research involves the generation of knowledge with specific, practical objectives. China’s applied research expenditures increased from 15.9 percent to 28.6 percent of the U.S. level and $13.2 billion to $39.9 billion over the course of the decade.
Experimental development is the use of existing knowledge to create or improve products and processes. China’s experimental development expenditures increased from $86.3 billion and 34.5 percent of the U.S. level in 2010 to $291.8 billion and 63.4 percent in 2020. Experimental development accounts for the majority of R&D expenditures for both China and the United States, though it accounted on average for 84 percent of R&D expenditures in China between 2010 and 2020 compared with 63 percent for the United States. As such, a slowdown in the growth of experimental development expenditures in China was responsible for the slowdown in overall R&D expenditures growth. These expenditures grew at an average rate of 17.3 percent per year in China between 2010 and 2015; this dropped to 8.8 percent in the second half of the decade.
R&D Intensity
Perhaps the most widely used indicator of a nation’s innovativeness, R&D intensity measures the extent to which a nation invests in R&D relative to its output.
Between 2010 and 2020, China’s R&D intensity increased from 1.7 percent to 2.4 percent, while the United States’ increased from 2.7 percent to 3.5 percent (figure 5). The two R&D intensities were converging in the first half of the decade but started to diverge after 2014 due to both increases in U.S. R&D intensity and a decrease in the growth rate of China’s.
Figure 6 shows China’s R&D components’ intensity relative to the United States’. At its relative peak in 2014, China’s R&D intensity was 74.4 percent of the U.S. level (2.0 percent in China and 2.7 percent in the United States).
China’s basic research intensity relative to the United States was much lower than its general R&D intensity at just 28 percent of the U.S. level in 2020. However, unlike general R&D intensity, China’s basic R&D intensity grew relative to the United States throughout the previous decade, increasing from 15.8 percent of the U.S. level in 2010.
In contrast to basic research intensity, China’s 2020 applied research and experimental development intensities relative to the United States were little changed from their 2010 figures. China’s applied research intensity was approximately 40 percent of the U.S. level in both 2010 and 2020, and its experimental development intensity increased from 85.4 percent of the U.S. level in 2010 to 90.1 percent in 2020. However, China’s experimental development intensity relative to the United States’ fell from its decade-high of 101.9 percent of the U.S. level in 2016 due primarily to faster growth in U.S. experimental development intensity. China’s relative applied research intensity remained roughly constant throughout the decade.
R&D Expenditures by Performing Sector
Another way to categorize R&D expenditures is by the sector performing the activity: business, government, or higher education. As can be seen in figure 7, China’s expenditures on R&D activities performed by the government or higher education institutions increased steadily relative to the United States’. China’s expenditures on business R&D relative to the United States’ was stagnant over the second half of the decade. China’s business-performed R&D expenditures increased from $76.6 billion in 2010 to $270.2 billion in 2020, which represented 27.5 percent and 49.8 percent of the U.S. levels, respectively. However, the average annual growth of such expenditures in China slowed from 17.9 percent per year in the first half of the decade to 9.1 percent in the second (and increased from 5.7 percent to 8.1 percent in the United States). China’s expenditures on R&D performed by the government approached U.S. levels by the end of the decade. Such expenditures in China increased from $18.9 billion (36.3 percent of the U.S. level) to $55.5 billion (81.5 percent of the U.S. level). Lastly, China’s expenditures on R&D performed by higher education institutions increased from $8.8 billion in 2010 to $27.2 billion in 2020; these figures represented 14.6 percent and 33.6 percent of the U.S. level, respectively.
R&D Intensity by Performing Sector
China’s R&D intensity relative to the United States’ varies significantly depending on which performing sector is being examined, as can be seen in figure 8. As with general R&D, China’s business-performed R&D intensity relative to the United States’ increased over the previous decade—from 67.9 percent to 70.7 percent of the U.S. level—but was also subject to a decrease from its mid-decade peak (of 80.5 percent in 2014). China’s government- and higher-education-performed R&D intensities relative to the United States’ increased throughout the previous decade from 89.7 percent to 115.8 percent and from 36.1 percent to 47.7 percent of U.S. levels, respectively. China’s government-performed R&D intensity increased from 0.31 percent of GDP in 2010 to 0.38 percent in 2020 (compared with 0.35 percent and 0.33 percent, respectively, in the United States), and its higher-education-performed R&D intensity increased from 0.14 percent of GDP to 0.19 percent (compared with 0.4 percent and 0.39 percent, respectively, in the United States). Given the scope and involvement of the Chinese government in economic and social affairs—especially the government’s promotion of “indigenous innovation”—it is unsurprising that government-performed R&D intensity is now approximately 16 percent greater in China than in the United States, although this relative growth stalled in the second half of the decade.
It may at first seem surprising that China’s basic research intensity is so low relative to the United States given its greater government-performed R&D intensity and attention government R&D places on basic research. However, business-performed R&D expenditures make up the greatest share of total R&D expenditures by far, accounting for 77 percent of China’s R&D expenditures and 75 percent of the United States’ R&D expenditures in 2020.46 Moreover, applied research expenditures make up a much larger share of business-performed R&D expenditures in the United States than in China, accounting for 6.4 percent of business-performed R&D expenditures in the United States in 2020, compared with only 0.5 percent in China.47 Lastly, while China’s government-performed R&D intensity is greater than the United States’, the U.S. government actually supplies a greater amount of R&D funding, both in absolute terms and relative to GDP.48
Top Firms Globally by R&D Investment
The EU Industrial R&D Investment Scoreboard lists the 2,000 companies with the greatest R&D investments globally (although it was only 1,400 in 2010 and 2011 and 1,500 in 2012). While the United States’ large companies maintained footholds at the top of the list throughout the decade, China made significant advancements both in terms of the number of companies in the top 1,400 and in the cumulative R&D investments of those companies.
In 2010, China had just 19 companies ranked in the top 1,400, which was 3.9 percent of the United States’ 487 (figure 9). China’s highest-ranking company was Huawei Technologies at 56. By 2020, China had 278 companies in the top 1,400, 61.9 percent of the United States’ 449. Huawei remained China’s highest-ranking company and jumped to second, behind the United States’ Alphabet. The cumulative R&D investment of China’s companies in the top 1,400 increased from 4.8 percent of U.S. levels in 2010, to 16.9 percent in 2015, and then to 38.1 percent in 2020.
Venture Capital
VC involves private investors financing small, risky business ventures (typically related to technology) in exchange for equity in the company. VC played a large role in the tech boom in the United States, helping now-household names such as Alphabet and Apple establish themselves as corporate giants and providing the funds needed to innovate in their early stages. VC has long been a more common source of financing in the United States than elsewhere in the world, but it is gaining traction worldwide—especially in China—and developing a relevant VC industry was a point of emphasis in MLP.50
Total VC Investments
China’s VC investments relative to the United States’ doubled over the previous decade from 23.2 percent to 46.6 percent of U.S. levels (figure 10), with China’s VC investments totaling $60.2 billion in 2020. However, this is down from its absolute peak of $74.8 billion in 2018 and its relative peak of 87.9 percent of U.S. levels in 2016. Nonetheless, China was still the second-largest VC market in the world in 2020, and VC continues to become a more instrumental part of China’s financial framework.51
VC Investments as a Share of GDP
Figure 11 shows China’s VC investments as share of GDP relative to the United States’. In 2010, China’s VC investments were 0.02 percent of its GDP, half of the United States’ 0.04 percent. By 2020, China’s VC investments increased to 0.4 percent of its GDP, while the United States’ VC investments increased to 0.62 percent of GDP. The mid-decade surge in China’s VC ecosystem relative to the United States’ is even more pronounced. Between 2013 and 2016, VC as a share of China’s GDP increased from 0.02 percent to 0.26 percent, while the United States’ only increased from 0.07 percent to 0.18 percent.
Researchers
While R&D intensity is a measure of the share of an economy’s resources it devotes to the creation of knowledge and novel products and processes, the number of researchers as a share of total workers measures the relative amount of human capital devoted to these activities. In this regard, China lags much further behind the United States, although it made steady progress throughout the decade.
Total Researchers
Figure 12 shows China’s number of total researchers relative to the United States’. China entered the decade with over 9 percent more researchers than did the United States (1.21 million compared with 1.11 million). This increased throughout the decade, and in 2019, China employed 2.17 million researchers, which was 44.5 percent more than the United States’ 1.5 million.
Researchers as a Share of Total Employees
Measuring researchers as a share of total employed workers reveals the relative amount of human capital devoted to core R&D activities. In this regard, China performs much more poorly than the United States. In 2010, there were 1.6 researchers per 1,000 employees in China compared with 8.5 in the United States. By 2019, this increased to 2.8 for China and 9.9 for the United States. While China’s concentration of researchers increased relative to the United States’ throughout the decade, increasing from 18.8 percent to 28.2 percent of the U.S. level (figure 13), China’s researchers as a share of total employees relative to the United States was still far below its R&D intensity relative to the United States at the end of the decade. A potential reason for this is that R&D incentives from the Chinese government (financial, political, etc.) cause labs and businesses to inflate their reported R&D expenditures. Another reason is that China’s R&D personnel are much more concentrated in non-research roles such as technician or administrator. In 2020, researchers made up just 44 percent of China’s R&D personnel.55 While comparative figures are not available for the United States, researchers make up 70–80 percent of R&D personnel in countries such as Canada, Japan, and South Korea.
Undergraduate Degrees Awarded
Degrees awarded measures the extent to which students with advanced skills for an innovation-driven and modern economy are added to the workforce. This measure is likely less representative for China than for the United States given the rate at which students from the former country travel abroad (often to the latter country) for their university educations, with approximately four-fifths of them returning to China after graduation.57 However, it is still useful for gauging the education and skill attainment of the populations.
Total Undergraduate Degrees Awarded
Figure 14 shows the number of both total and science and engineering undergraduate degrees awarded in China relative to the United States. China already awarded 56.8 percent more total degrees and 106.5 percent more science and engineering degrees than did the United States in 2010. These both increased throughout the decade, and although both finished slightly below their decade highs, by 2018, China awarded 94.7 percent more total undergraduate degrees and 124.6 percent more science and engineering undergraduate degrees than did the United States. Specifically, China awarded 3.87 million total undergraduate degrees to the United States’ 1.99 million and 1.82 million science and engineering undergraduate degrees to the United States’ 0.81 million.
Figure 15 considers individual subject groups: natural sciences, mathematics, and statistics as one group and computer science and engineering as another. China entered the decade awarding almost twice as many undergraduate degrees in natural sciences, mathematics, and statistics as did the United States (194.4 percent as many). However, by 2018, this dropped to only 29 percent more, and China awarded 13,500 fewer such degrees than it did in 2010. The largest relative drop was between 2012 and 2013, when China’s number of such degrees awarded fell by over 15 percent.
China awarded 469.9 percent more computer science and engineering undergraduate degrees than did the United States in 2010. By 2014, this increased to 538.5 percent more. However, the United States started catching up to China after 2014, and by 2018, China awarded “only” 444.9 percent more such degrees than did the United States. This drop was the result of a slowdown in the growth of such degrees in China (from 8.6 percent per year in 2010–2014 to 2.9 percent in 2014–2018) while the United States’ growth rate increased (from 5.6 percent per year in 2010–2014 to 7.1 percent in 2014–2018).
Undergraduate Degrees Awarded per Capita
It is also helpful to account for the size of the populations being considered. Only a narrow age range accounts for the vast majority of undergraduate university students. Thus, the number of undergraduate degrees awarded per capita of each country’s 20–29-year-old population is considered. Although China’s age distribution is more skewed toward the older end of the distribution than is the United States’ in general, the 20–29 age group makes up a larger share of its population.60 While this was true throughout the decade, that share fell quite rapidly. The age group’s share of the population in China fell from about 17 percent in 2010 to 14 percent in 2018.61 In the United States, the share remained constant at approximately 14 percent.62 Disregarding these dynamics therefore understates China’s progress, as can be seen by comparing figure 14 and figure 15 with figure 16 and figure 17.
Total undergraduate degrees awarded per capita in China increased from 29.5 percent of U.S. levels in 2010 to 44.3 percent in 2018 when it awarded 195 such degrees for every 10,000 residents in the age group (figure 16). For science and engineering fields specifically, the increase was from 38.9 percent to 51.1 percent of U.S. levels over that period, with China awarding 92 such degrees per 10,000 residents in the age group in 2018.
Relative growth was negative over the decade for China when looking specifically at natural sciences, mathematics, and statistics degrees, falling from 36.5 percent of the U.S. level in 2010 to 29.3 percent in 2018 (figure 17). These represent approximately 12 and 13 such degrees being awarded per 10,000 residents in the age group in China those years.
Entering the decade, China already awarded 35 computer science and engineering undergraduate degrees per 10,000 residents in the age group, which amounted to 7.3 percent more than the United States. China added to this lead throughout the decade, awarding 64 degrees per 10,000 in 2018, 23.9 percent more than the United States’ 52. Relative growth slowed in the second half of the decade, but 123.9 percent of the U.S. level marked China’s decade high.
Figure 16: Undergraduate degrees awarded per capita in China relative to the United States (20- to 29-year-old populations)63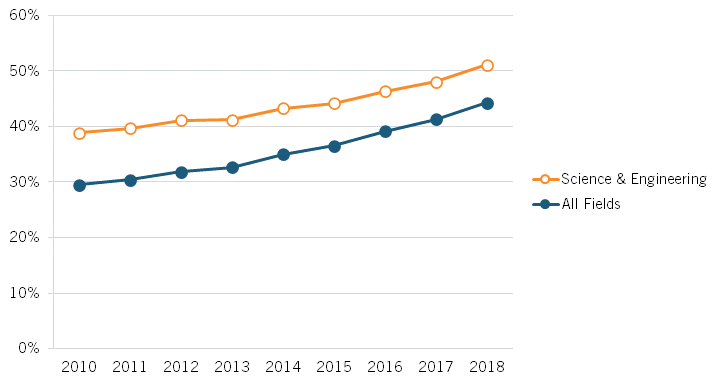
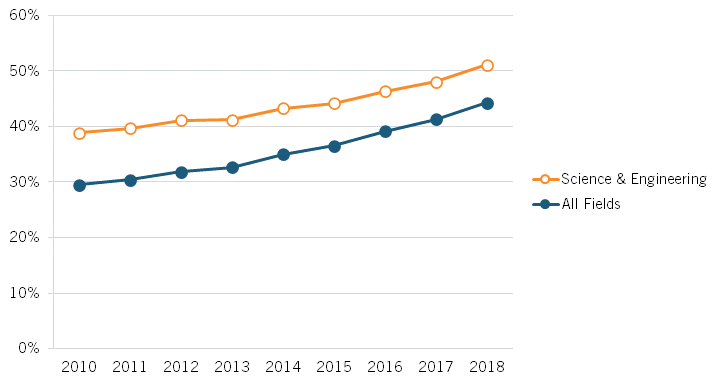
Figure 17: Science and engineering undergraduate degrees awarded per capita in China relative to the United States (20- to 29-year-old populations)64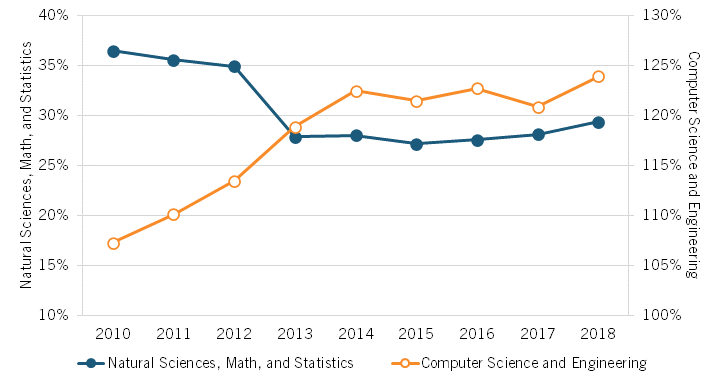
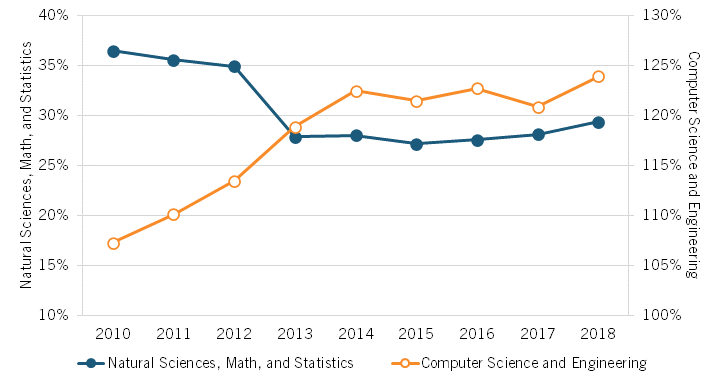
Doctoral Degrees Awarded per Capita
Considering doctoral degrees rather than undergraduate degrees shifts the focus to students with advanced education and skills in their respective fields who have demonstrated the ability to conduct research at a high level. Again, many Chinese nationals go abroad for their advanced education. To the extent a nonnegligible proportion of these students return to China after receiving their degrees, this indicator understates the number of new PhDs entering China’s workforce each year. However, there is reason to believe that this is much less understated than undergraduate degrees. Despite progress in developing and retaining researching talent, a Center for Security and Emerging Technology study from earlier this year found that 90 percent of Chinese nationals who received a STEM PhD in the United States between 2000 and 2015 remained in the country as of February 2017.65
Total Doctoral Degrees Awarded
Unlike with undergraduate degrees, China did not award more doctoral degrees than did the United States entering the decade or at any time between 2010 and 2018 (figure 18). This is also true for science and engineering doctoral degrees. In 2010, China awarded 85.3 percent as many total doctoral degrees as did the United States. By 2015, this had fallen to 78 percent as the United States’ growth rate of such degrees outpaced China’s. However, this trend reversed, and China awarded 83.5 percent as many total doctoral degrees as did the United States in 2018. That same year, China awarded 60,700 doctoral degrees to the United States’ 72,700.
A similar dynamic played out for science and engineering degrees. China awarded 93.3 percent as many such degrees as did the United States in 2010. By 2014, this fell to 85.9 percent. As with total degrees, this trend reversed, and by 2018, China produced 96.8 percent as many science and engineering doctoral degrees as the United States (39,800 to 41,100).
Figure 18: Number of science and engineering doctoral degrees awarded in China relative to the United States66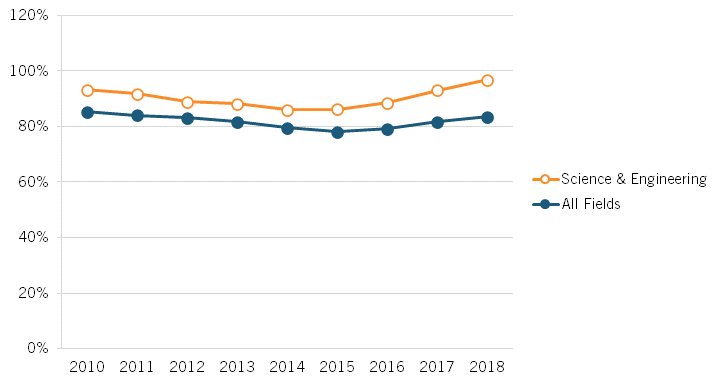
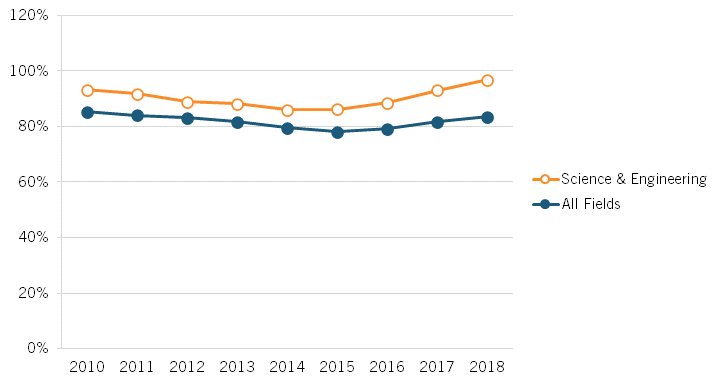
In contrast to undergraduate degrees, total doctoral degrees, and science and engineering doctoral degrees in general, China’s number of doctoral degrees awarded in the natural sciences, mathematics, and statistics relative to the United States’ increased steadily throughout the decade, from 65.2 percent to 75.1 percent of the U.S. level (figure 19). During the decade, China’s growth rate of such degrees was twice that of the United States (3.6 percent per year versus 1.8 percent per year).
China entered the decade awarding over 80 percent more computer science and engineering doctoral degrees than did the United States. The relative dynamics for these degrees were much more like those for total doctoral degrees and science and engineering doctoral degrees in general. From 2010 to 2015, China’s awarding of such degrees dropped from 182.8 percent to 148.8 percent of the U.S. level. However, again, China’s growth rate of such degrees picked up while the United States’ slowed down. In 2018, China awarded 67.3 percent more computer science and engineering doctoral degrees than did the United States, which was still below its 2010 level.
Figure 19: Number of science and engineering doctoral degrees awarded by subject group in China relative to the United States67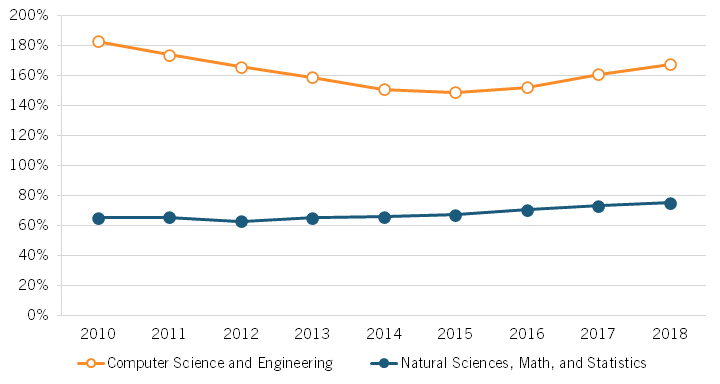
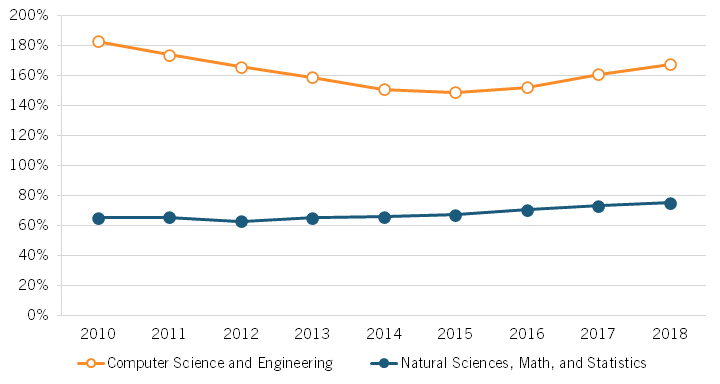
Doctoral Degrees Awarded per capita
For analysis of doctoral degrees per capita, the countries’ 25–39-year-old populations were considered. Among doctoral recipients in the United States, 88 percent are 40 years old or younger—and this concentration is greater for science and engineering fields.68 Again, this age group accounted for a larger share of the population in China than in the United States throughout the decade (over 23 percent in China and under 21 percent in the United States in 2018).69 However, changes in the groups’ population shares in the two countries were very similar, so only comparing total degrees does not have the same progress-understating effect that it does for undergraduate degrees.
China’s doctoral degrees awarded per capita relative to the United States are much lower than for undergraduate degrees in all categories. In 2010, China awarded 16.9 percent as many doctoral degrees per 25–39-year-old resident as did the United States (figure 20). In 2018, this was unchanged, although it was up from the decade low of 15.1 percent in 2015. The story of China’s science and engineering degrees per capita relative to the United States is similar. In 2010, China awarded 18.5 percent as many such degrees per age-group resident as did the United States. By 2018, this increased slightly to 19.7 percent. Here, too, China reversed a negative trend that defined the first half of the decade.
Figure 20: Number of doctoral degrees awarded per capita (25–39-year-old population) relative to the United States, 2010–201870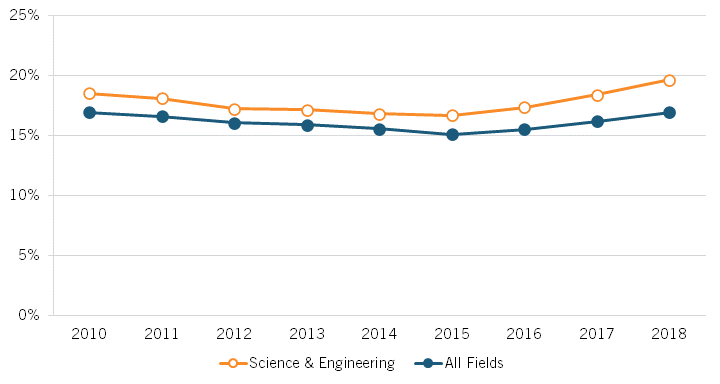
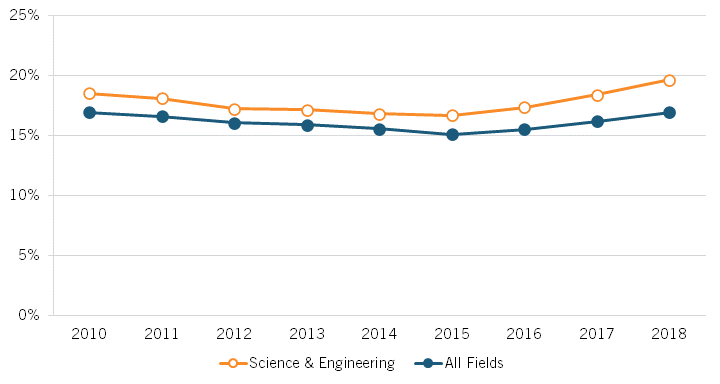
As with science and engineering fields as a whole, China’s natural sciences, mathematics, and statistics doctorates awarded per 25–39-year-old relative to the United States rose only slightly throughout the decade, from 12.9 percent of the U.S. level in 2010 to 15.3 percent in 2018 (figure 21). However, China’s progress in this subject group was much steadier. The dynamics for computer science and engineering doctorates per capita are much more like those for total degrees and science and engineering degrees as a whole. Between 2010 and 2015, China’s computer science and engineering doctorates awarded per capita fell from 36.3 percent of the U.S. level to 28.8 percent. By 2018, this recovered somewhat to 33.9 percent.
Figure 21: Science and engineering doctoral degrees awarded per capita in China by subject group relative to the United States (25- to 39-year-old population)71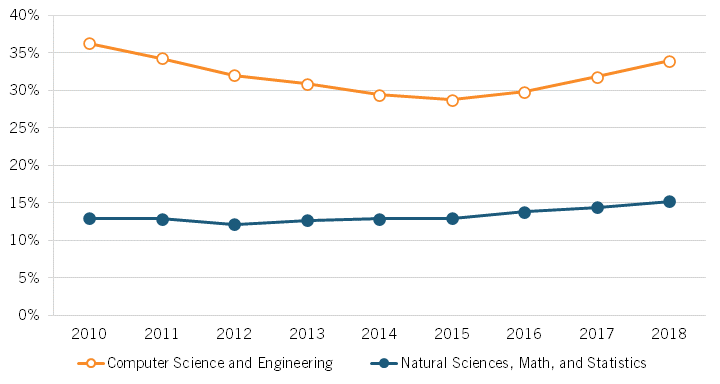
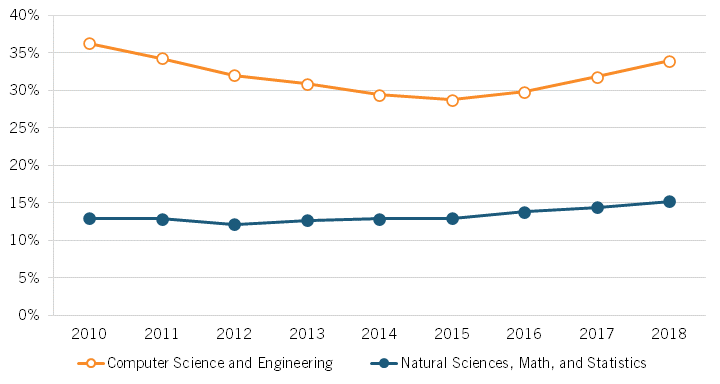
Top Universities Globally
As the institutions that train future high-skill workers and researchers—and among the primary contributors to the accumulation of foundational knowledge—the number and quality of top universities in a country are useful indicators of its innovative capacity.
Per the annual Shanghai Academic Ranking of World Universities, China had 27 universities in the top 500 in 2010 compared with the United States’ 154 (including all of the top four). By 2020, China’s number of universities in the top 500 jumped to 76, whereas the United States’ number dropped to 133 (although it now held all top five spots). Moreover, China caught up to the United States at a faster pace in the second half of the decade.
Weighted scores were given to universities based on their standings in the rankings to account for both the number and relative quality of the universities in the top 500. The appendix in this report provides an explanation of this scoring method. The cumulative value of the weighted scores of China’s universities in the top 500 was only 9 percent that of the United States’ universities (figure 22). In 2020, China’s cumulative score was 40.5 percent that of the U.S. score. As figure 22 shows, China’s progress in both the number of universities and their cumulative weighted score relative to the United States was exponential.
Summary of Innovation Inputs
In the last decade, China gained ground relative to the United States in almost every input indicator reviewed. However, it still lags behind the United States in all indicators accounting for size. China made significant strides with respect to its top R&D-investing companies and the number and quality of its top universities; progress relative to the United States was exponential for both. While still far behind U.S. levels, China made steady progress in basic R&D intensity and researchers as a share of its employed workforce. China briefly caught up to the United States in terms of VC investment in the middle of the decade (and surpassed the United States when accounting for the size of the economy) but fell back down to about half of U.S. VC investment in 2020. Nevertheless, VC is becoming an increasingly integral part of the Chinese financial sector. While China now produces the most university graduates of any country, its number of graduates per capitaremains well below the comparative U.S. figures in almost all fields reviewed.73 For both undergraduate and doctoral degrees per capita in the natural sciences, mathematics, and statistics, China is still far behind the United States and struggling to make progress.
INNOVATION OUTPUTS
Science and Engineering Articles
A nation’s science and engineering research articles measures the extent to which that nation contributes to the building of knowledge in these innovation-related subjects. The following figures show China’s output of science and engineering articles, both as a whole and by subject, relative to the United States.
Total Science and Engineering Articles
Over the last decade, China went from publishing 68.7 percent as many science and engineering articles as did the United States in 2010 to 123.7 percent in 2020, publishing over 742,000 articles compared with the United States’ 600,000 (figure 23). Moreover, China caught up (and then pulled away) at an increasing rate throughout the decade. In 2010–2015, China’s science and engineering publications grew at an annual rate of 6 percent compared with the United States’ 1.9 percent. In 2015–2020, China’s rate increased to 10.7 percent per year while the United States’ only increased to 2.3 percent.
Figure 24 shows China’s technical sciences (computer and information sciences, engineering, and mathematics and statistics) publications relative to the United States. For computer and information sciences and engineering, China entered the decade already publishing more articles per year than the United States. In the first half of the decade, China’s computer and information sciences publications fell at an annual rate of 9.1 percent and its engineering publications increased at an annual rate of 5.8 percent. In the second half of the decade, these rates increased to (positive) 14.4 percent and 8.7 percent, respectively. The comparative increases for the United States were much smaller: 0.6 percent to 1.8 percent for computer and information sciences and 0.4 percent to 0.5 percent for engineering. China’s second-half progress in mathematics and statistics publications was more muted. China’s publications in these subjects increased at an annual rate of 10.1 percent in the first half of the decade while the United States’ increased at 2.6 percent. In the second half of the decade, China’s annual growth rate fell slightly to 8.8 precent while the United States’ rose to 3.3 percent.
Figure 24: Number of technical sciences articles in China relative to the United States, by subject75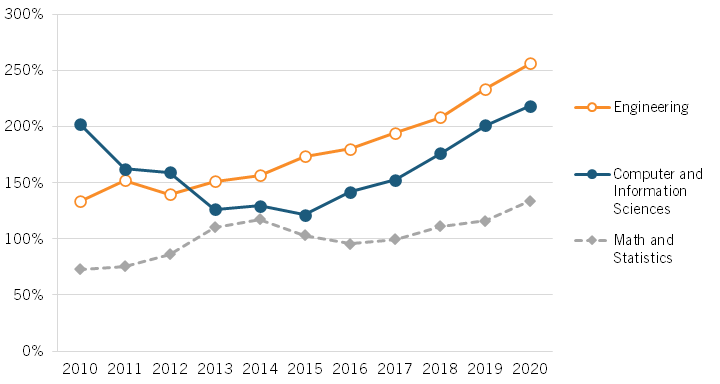
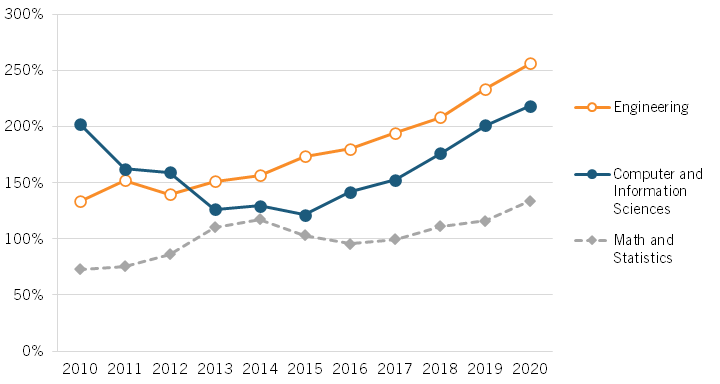
Figure 25 focuses on the natural sciences. China entered the decade out-publishing the United States in only chemistry, though by the end of the decade, it published 3.5 times as many articles as the United States in chemistry, 2.5 times as many in physics, and 12.8 percent more in biological and biomedical sciences. In contrast to the technical sciences, China’s annual growth rate in publication output increased from the first to the second half of the decade in only physics (7.1 percent to 10 percent). This was true for the United States for only geological, atmospheric, and oceanic sciences (2.1 percent to 3 percent), but the decreases in the United States’ annual growth rates in biological and biomedical sciences (1.2 percent to 0.5 percent) and chemistry (0.9 percent to -0.21 percent) were smaller than China’s (13.3 percent to 11.9 percent and 11.4 percent to 6.6 percent, respectively). Hence, while China still made progress relative to the United States in biological and biomedical sciences, chemistry, and geological, atmospheric, and oceanic sciences in the second half of the decade, it did so at a slower pace.
Science and Engineering Articles per Capita
Again, accounting for the size of China’s population provides a clearer picture. Because China’s population was approximately 4.3 times larger than that of the United States throughout the decade, per capita progress closely matched progress when considering total measures. Over the last decade, China went from publishing 15.9 percent of the United States’ science and engineering articles per capita in 2010 to 29.1 percent in 2020 (figure 26), when it published 523.2 science and engineering articles per million residents compared with the United States’ 1,810.1.
Figure 26: Number of science and engineering articles per capita in China relative to the United States77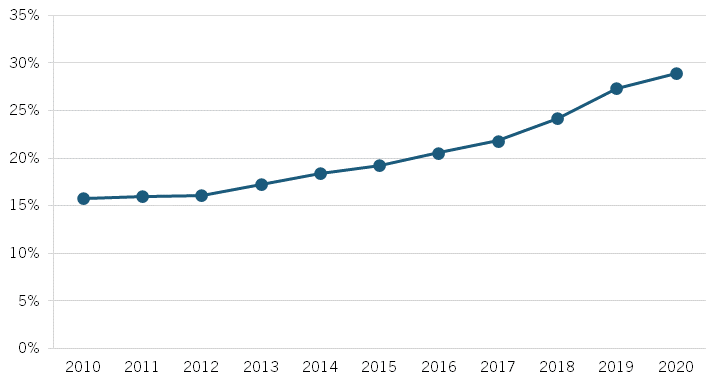
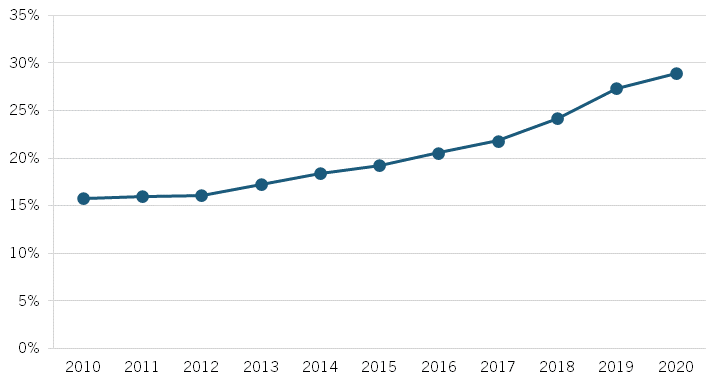
Figure 27 shows China’s number of articles per capita in the technical sciences relative to the United States by subject. China produced fewer than half as many articles per capita than did the United States in each of the three categories in 2010 (and less than 20 percent as many in mathematics and statistics). This increased for each subject, though China only produced 59.9 percent as many engineering articles, 51.1 percent as many computer and information sciences articles, and 31.1 percent as many mathematics and statistics articles per capita than did the United States in 2020. China produced 122.7, 62.5, and 12.6 articles in these subjects per million residents, respectively, in 2020.
Figure 27: Number of technical sciences articles per capita in China relative to the United States, by subject78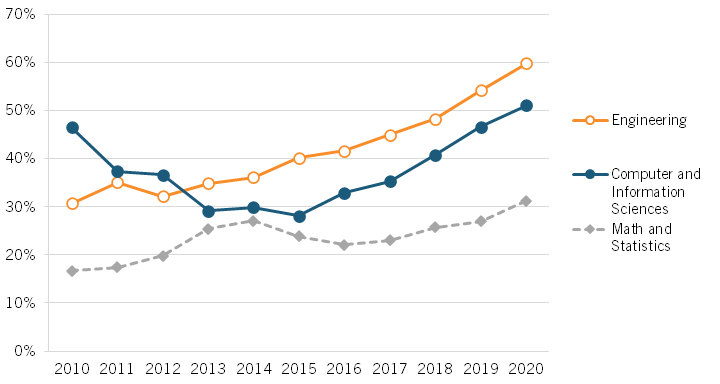
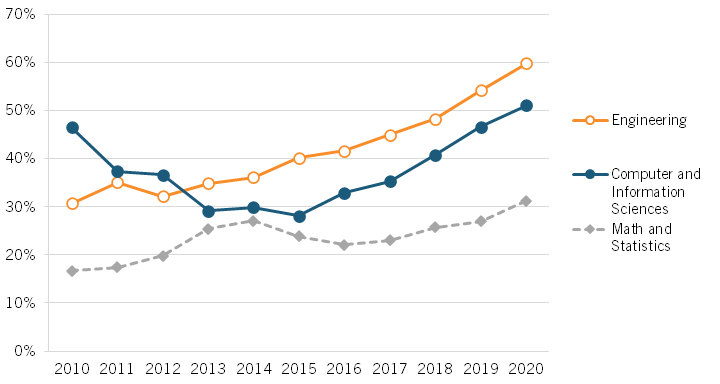
Figure 28 shows the relative per capita figures for the natural sciences. Again, China produced fewer than half as many articles per capita as did the United States in each subject entering the decade (and less than 10 percent as many in the biological and biomedical sciences and the geological, atmospheric, and oceanic sciences). China experienced consistent growth relative to the United States in each of the subjects throughout the decade, and China’s per capita output of chemistry articles approached the U.S. level by the end of that period. In 2020, China produced 83.1 percent as many articles per capita as did the United States in chemistry; 58.7 percent as many in physics; 26.4 percent as many in the biological and biomedical sciences; and 14.4 percent as many in the geological, atmospheric, and oceanic sciences. China produced 39.2, 47.6, 51.8, and 72.7 articles per million residents in these subjects, respectively, in 2020.
Figure 28: Number of natural sciences articles per capita in China relative to the United States, by subject79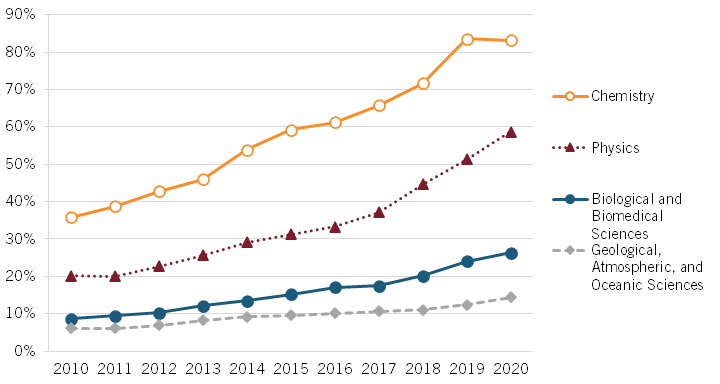
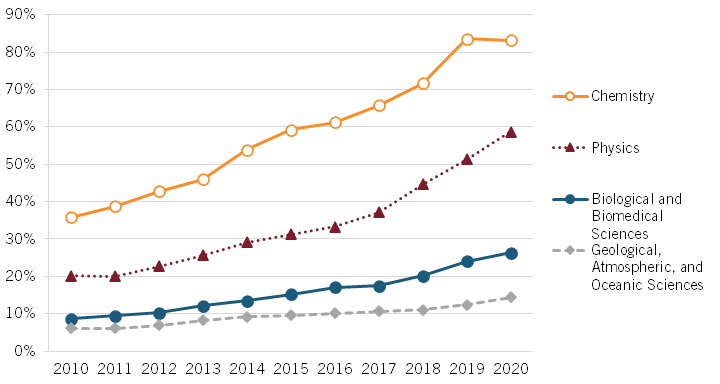
Top-Cited Science and Engineering Articles
Beyond the sheer volume of a nation’s science and engineering articles published, it is useful to gauge the quality of those articles. One way to do this is by measuring what share of that nation’s articles are among the most cited in their fields. The following figures compare what shares of China’s science and engineering articles are among the top 1 percent in citations in the two years following their publications. By definition, the global (weighted) average is 1 percent. In 2010, only 0.5 percent of China’s science and engineering articles were among the most cited, compared with 1.9 percent for the United States (figure 29). By 2018, however, China attained the global average of 1 percent while the share of the United States’ science and engineering articles among the most published dropped to 1.6 percent. This means China’s science and engineering articles were approximately 60 percent as likely to be among the top 1 percent in citations as were U.S. articles in 2018.
Figure 29: Share of Chinese science and engineering articles ranking among the top 1 percent globally in citations relative to the United States80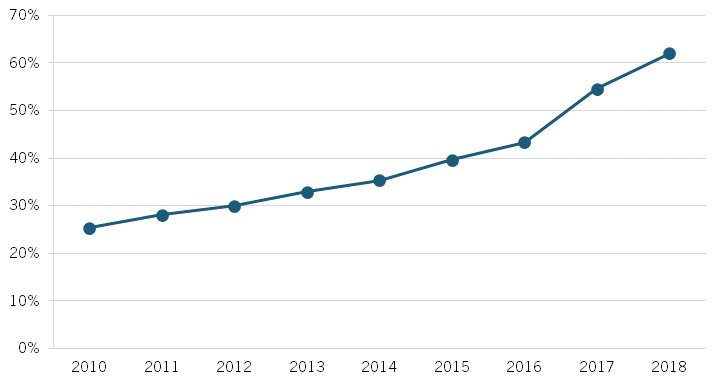
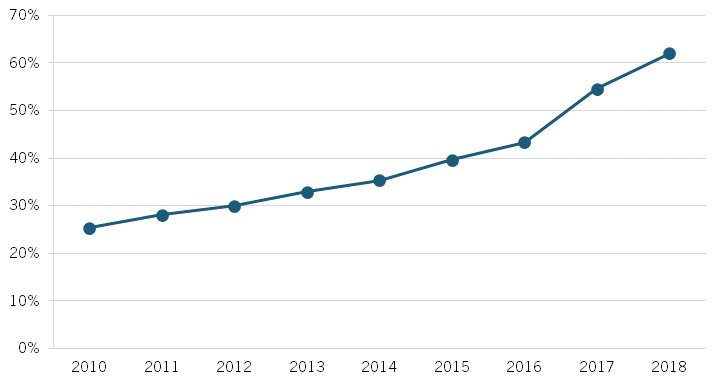
Looking at individual science and engineering subjects, we see that the gains in influence relative to U.S. articles were universal (figure 30 and figure 31). The biggest relative gains made were in mathematics and statistics (where Chinese articles were 68 percent more likely to be among the top 1 percent in citations than their American counterparts in 2018) and chemistry (where Chinese articles were only 11.3 percent less likely to be among the most cited in 2018). China came close to U.S. levels of influence in engineering research, as China’s engineering publications were 74.6 percent as likely as their American counterparts to be among the most cited in 2018.
Figure 30: Share of Chinese technical sciences articles ranking among the top 1 percent globally in citations relative to the United States, by subject81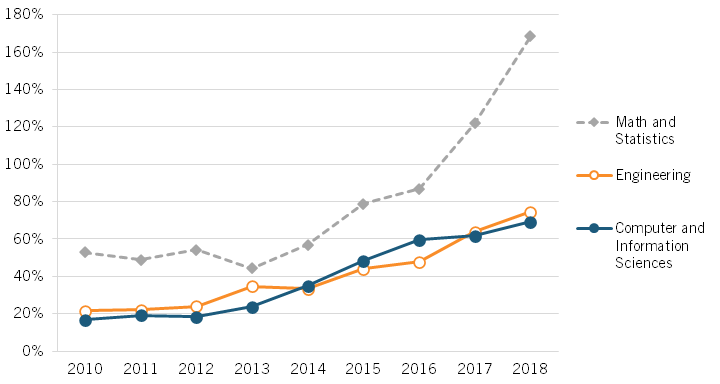
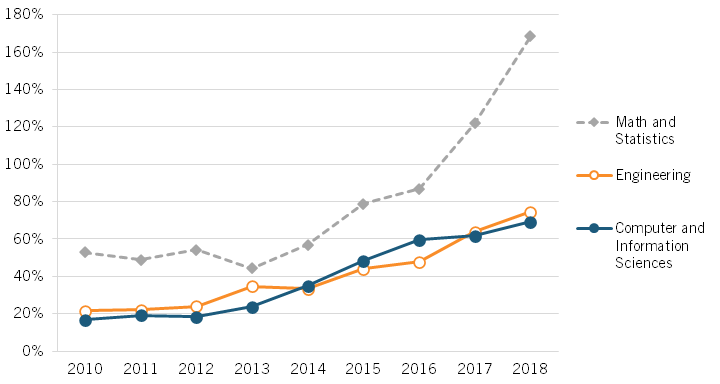
Figure 31: Share of Chinese natural sciences articles ranking among the top 1 percent globally in citations relative to the United States, by subject82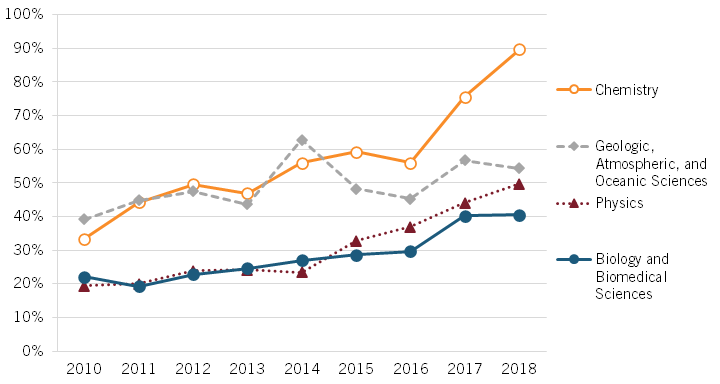
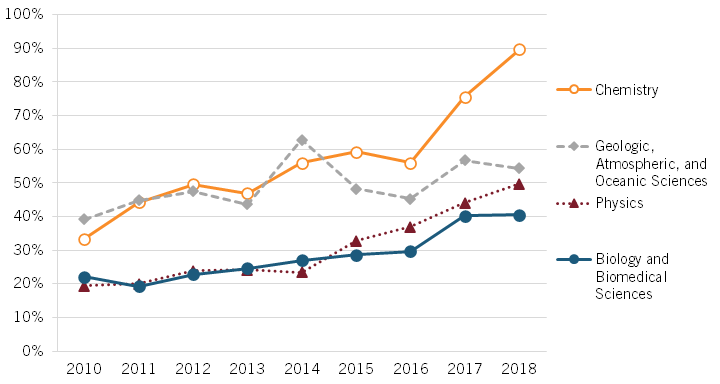
International Patent Families
An international patent family (IPF) is the collection of all patents covering the same or very similar inventions in multiple countries. As mentioned previously, patent activity in China can be somewhat misleading in the years following MLP in 2006, since the government’s encouragement of patent applications likely triggered an increase in applications for inventions that were not practical or economical.83 However, data regarding international patent families considers inventions for which patents were granted in several countries, which avoids counting patents for which there is no noteworthy difference between inventions as multiple patents and counting the likely less-valuable patents only filed in the inventor’s home country. While this does not completely erase the issue of China’s high proportion of relatively worthless patents (a Chinese patentholder can file a patent in another country with a cheap and easy patent process—and this measure does not differentiate between less-useful design and utility patents and more-useful invention patents), it does mitigate it and is preferable to a measure of patents filed with just the Chinese patent office or some other specific nation’s patent office.
Total IPFs
China entered the decade already receiving 9.4 percent more total IPFs per year than did the United States, and by 2020, it was awarded 378.4 percent more (figure 32). Over the course of the decade, China’s IPFs granted increased from 80,556 to 456,088 while the United States’ increased from 73,622 to 95,347. From 2010 to 2015, China’s IPFs granted increased at a staggering annual rate of 26 percent compared with the United States’ 4.3 percent. Growth slowed for both countries in the second half of the decade: China’s annual growth rate more than halved to 12.3 percent while the United States’ fell to 1 percent.
Figure 32: Number of international patent families granted to Chinese entities relative to the United States84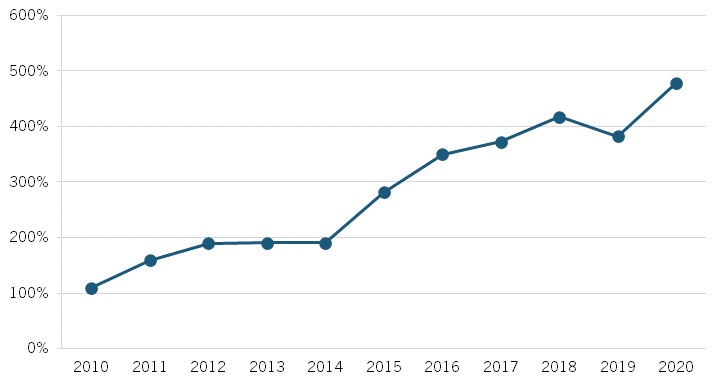
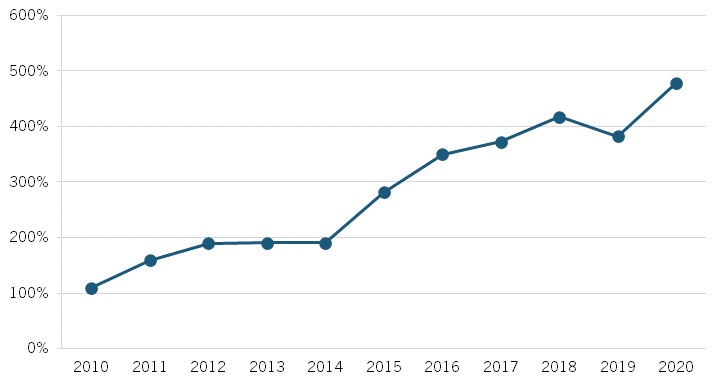
Two major objectives of MIC are to incorporate information and communications technologies (ICTs) into the manufacturing process to both increase productivity and become self-reliant with respect to the development of new and emerging technologies. While China’s respect for IP rights is significantly lacking, especially at the international level, IPFs granted to Chinese entities in these technologies provide a useful measure for the extent to which these goals are being achieved.
As shown in figure 33, China’s IPFs granted were fewer than those of the United States entering the decade in three of the four ICTs considered: telecommunications (77.7 percent of the U.S. level), computer technology (33.7 percent), and semiconductors (79 percent). By 2016, China surpassed the United States in all four ICTs. By the end of the decade, it received more than three times as many IPFs in each technology than did the United States. Moreover, China pulled away from the United States in the second half of the decade at a faster rate than it caught up in the first half for each ICT. In each technology except for semiconductors, this was a result of IPF growth rates increasing for China and falling for the United States. For semiconductors, IPF growth fell for both countries. However, for China, it only fell from 18.8 percent per year in 2010–2015 to 14.4 percent per year in 2015–2020. In the United States, the annual growth rate in semiconductor IFPs granted fell from 3.3 percent to -3.5 percent.
Figure 33: Number of ICT international patent families granted to Chinese entities relative to the United States, by field85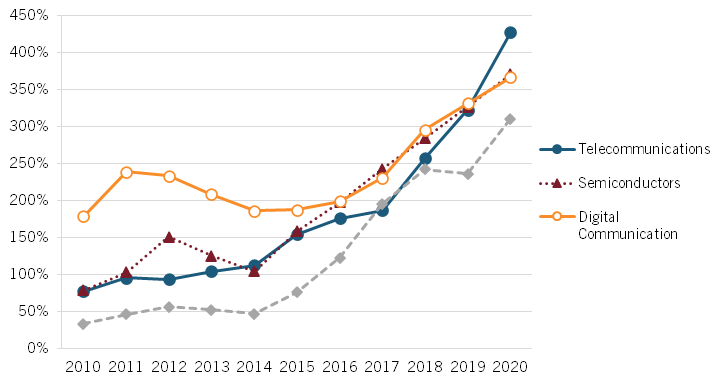
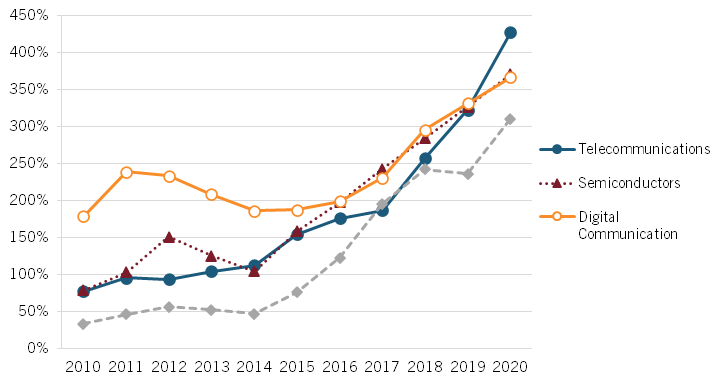
As with ICTs, MIC cites biopharmaceuticals/biotechnologies as an industry in which China aims to be a self-sufficient world leader and innovator. China entered the decade receiving more IPFs than did the United States in biotechnology (54.3 percent more) and pharmaceuticals (228.2 percent more), and it received only 38.5 percent as many medical technology IPFs, as seen in figure 34. China’s growth in medical technology IPFs relative to the United States was steady throughout the decade, and China was granted 74.6 percent more such IPFs than was the United States in 2020. Relative growth in biotechnology and pharmaceuticals was much more sporadic. In both technologies, China ceded ground to the United States during the middle of the decade due to significant decreases in China’s IPFs received per year. From 2013 to 2017, China’s IPFs awarded in biotechnology and pharmaceuticals dropped 24.8 percent and 33 percent, respectively. However, this quickly and significantly reversed. Between 2017 and 2020, China’s IPFs granted increased by 135.5 percent and 42.2 percent, respectively. Meanwhile, the United States’ biotechnology IPFs granted fell by 16.1 percent during that span. In 2020, China received approximately seven times as many biotechnology IPFs and over four times as many pharmaceuticals IPFs as did the United States.
Figure 34: Number of life sciences international patent families granted to Chinese entities relative to the United States, by field86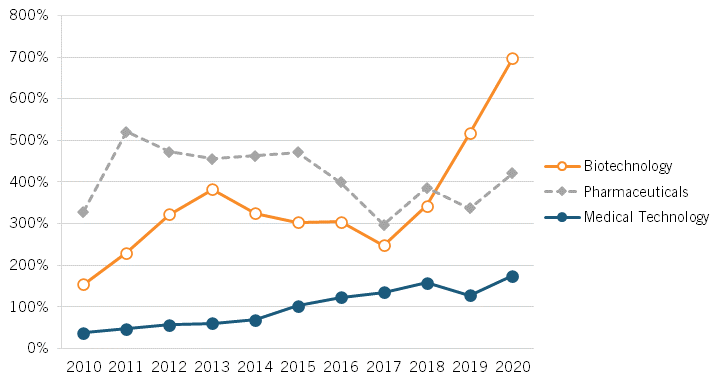
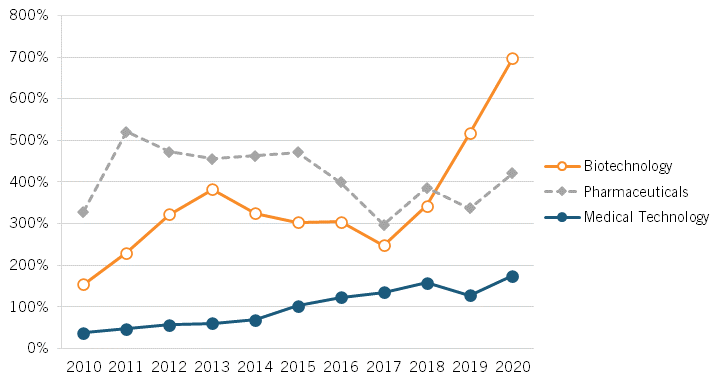
Environmental technologies (ETs), especially low-emission power-generating technologies, are paramount in a world that must rein in carbon emissions to confront global warming. These technologies are consistently included in China’s many lists of priority technologies. Specifically, MIC singles out new-energy motor vehicles as a technology in which China hopes to become a global leader. In 2010, China was already awarded twice as many ET IPFs as was the United States (figure 35). By 2020, this ballooned to 11.4 times as many despite two separate periods of little to no growth relative to the United States that account for half the decade (2012–2014 and 2016–2019). Between 2010 and 2015, China’s ET IPFs grew at a staggering 32.5 percent per year, compared with the United States’ 2.6 percent. These figures dropped to 8.8 percent per year and -0.7 percent per year for the two countries, respectively.
In the United States, the annual growth rate in semiconductor IPFs granted fell from 3.3 percent in the first half of the decade to -3.5 percent in the second half of the decade.
Figure 35: Number of enviro-tech international patent families granted to Chinese entities relative to the United States, 2010–202087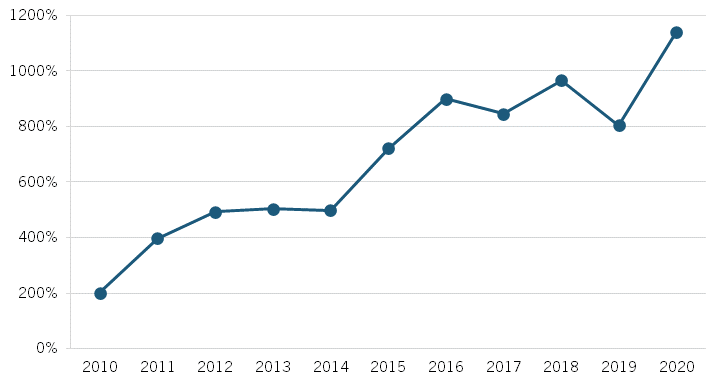
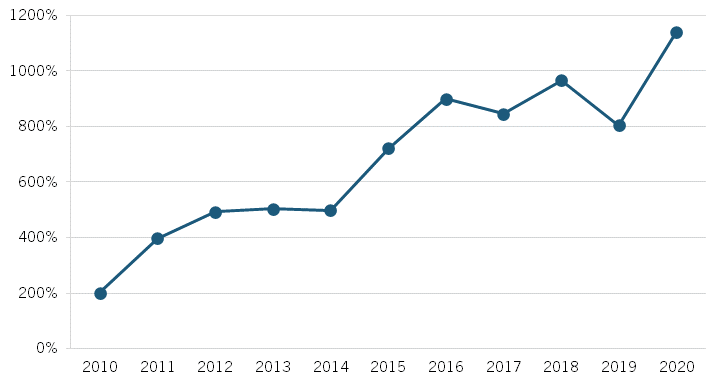
IPFs per Capita
Figure 36 shows China’s IPFs per capita relative to the United States’. China entered the decade only receiving one-quarter of the IPFs per capita the United States received (59.9 IPFs per million residents versus 238). However, by the end of the decade, China surpassed the United States in IPFs per capita: China received 321.4 IPFs per million residents in 2020, which was 11.7 percent more than the United States’ 287.6.
Figure 36: Number of international patent families granted to Chinese entities per capita relative to the United States88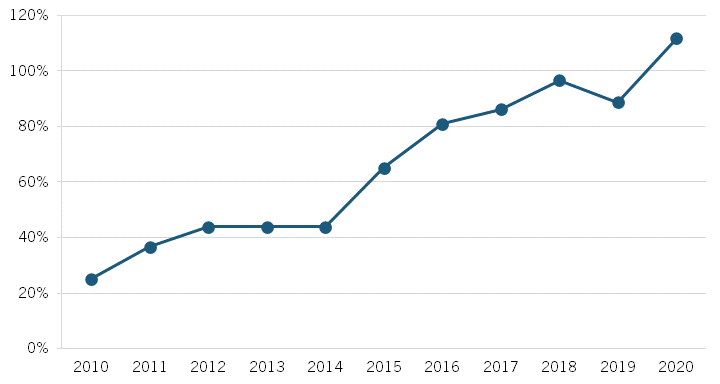
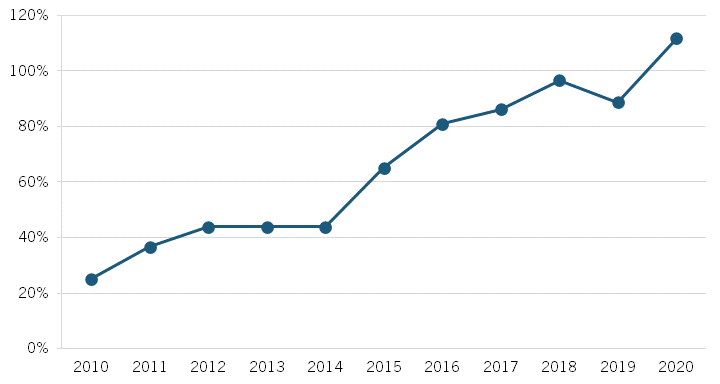
Figure 37 shows China’s ICT IPFs per capita relative to the United States’ broken down by field of technology. Again, China made significant progress in each technology. By 2020, China received more than 75 percent as many IPFs per capita as did the United States in each field and surpassed the United States with regard to telecommunications IPFs. Relative growth was faster in the second half of the decade than in the first for each of the four technologies.
Figure 37: Number of ICT international patent families granted to Chinese entities per capita relative to the United States, by field89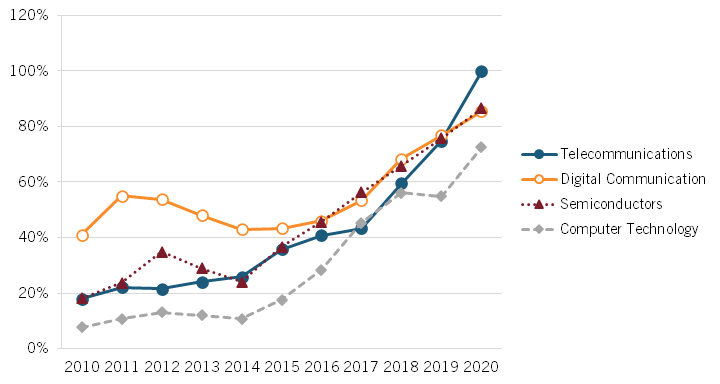
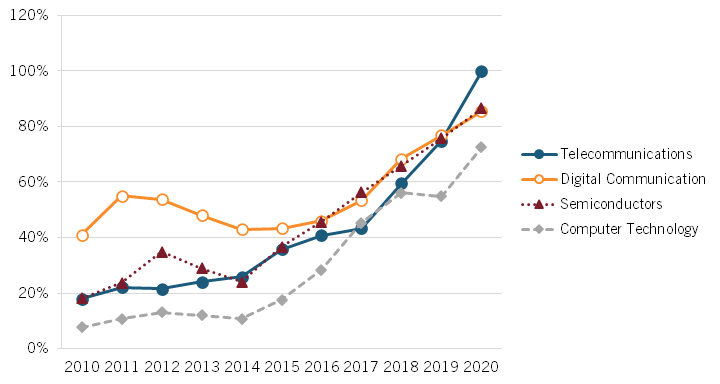
Figure 38 shows China’s life sciences IPFs per capita relative to the United States’ broken down by technology area. China’s progress was most significant in biotechnology, in which it received 62.8 percent more IPFs per capita than did the United States by the end of the decade. Though China made progress in each of the three areas, China’s pharmaceutical IPFs per capita relative to the United States finished the decade well below its peak of 119.8 percent of the U.S. level in 2011.
Figure 38: Number of life sciences international patent families granted to Chinese entities per capita relative to the United States, by field90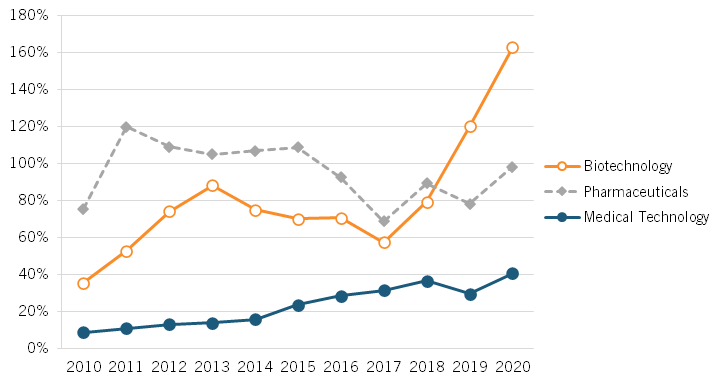
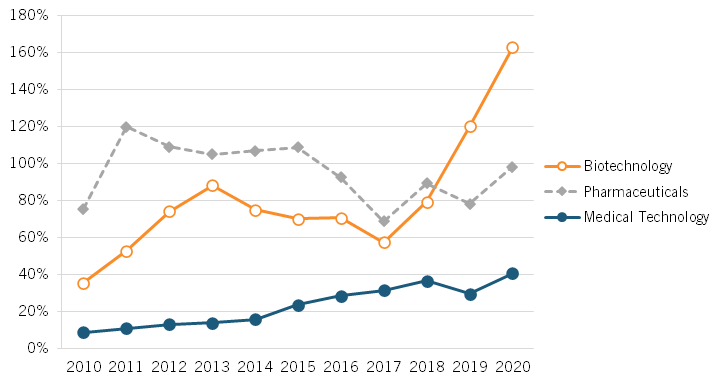
Figure 39 shows China’s ET IPFs per capita relative to the United States. China entered the decade producing fewer than half as many ET IPFs per capita as the United States. However, this quickly changed, as China surpassed the United States in 2012. China’s progress continued, and by the end of the decade, China produced 166.6 percent more ET IPFs per capita than did the United States.
Figure 39: Number of enviro-tech international patent families granted to Chinese entities per capita relative to the United States91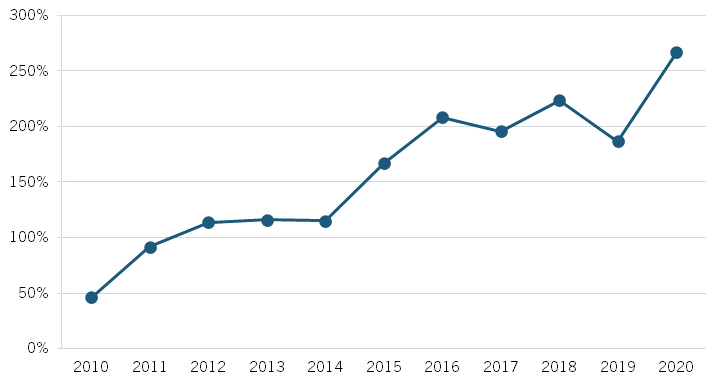
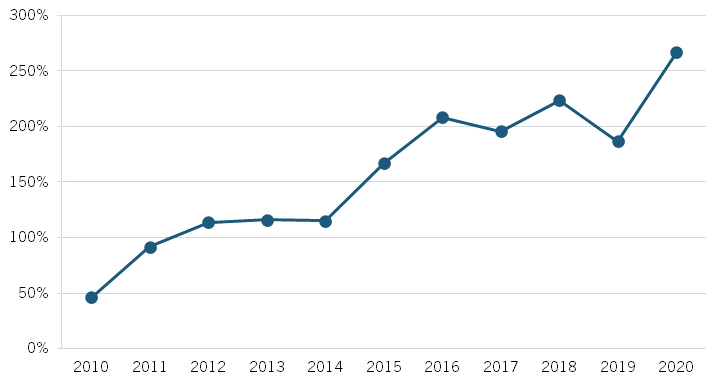
International IP Receipts
One way to measure the worth of patents granted is to measure the international licensing receipts Chinese patent and trademark holders receive. Cross-border receipts provide a measure of how valuable foreign entities view China’s IP relative to those of other countries.
Total International IP Receipts
As seen in figure 40, China receives very little in cross-border IP receipts compared with the United States, supporting the argument that China’s surge in patent filing is rather hollow. China’s $1.2 billion in international IP receipts in 2010 was only 1.3 percent of the United States’ $95 billion. For much of the decade, this remained the case. However, starting in 2016–2017, China’s international IP receipts increased at breakneck pace. Between 2016 and 2017 alone, China’s international IP receipts tripled from $1.8 billion to $5.4 billion, and by 2020, they were $9.3 billion. While this was still only 7.5 percent of the U.S. level ($113.8 billion), China’s progress—both in absolute and relative terms—is quite staggering.
Figure 40: Value of cross-border IP licensing receipts by Chinese entities relative to the United States92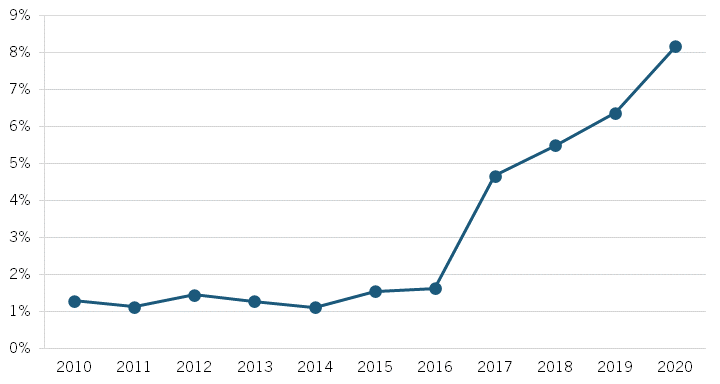
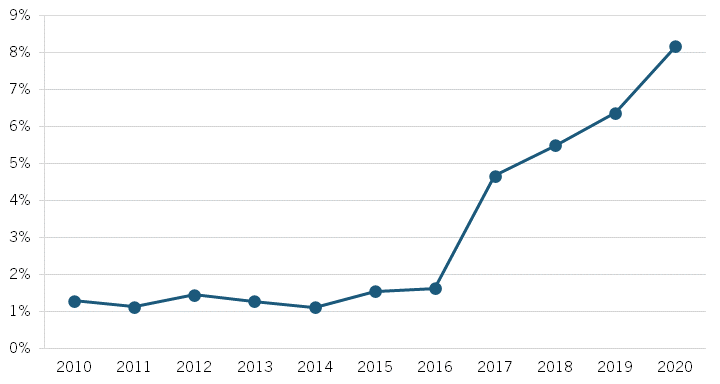
International IP Receipts as a Share of GDP
Taking these receipts as a share of GDP, as shown in figure 41, accounts for the size of each economy and measures the extent to which they specialize in producing IP for the competitive international market. In 2010, China’s international IP receipts accounted for only 0.02 percent of its GDP, compared with 0.63 percent for the United States. Again, China’s international IP receipts as a share of GDP remained miniscule relative to the United States’ before ballooning in 2017. By 2020, China’s receipts accounted for 0.06 percent of its GDP, which was 11.3 percent of the U.S. level.
Figure 41: Value of cross-border IP licensing receipts by Chinese entities as a share of GDP relative to the United States93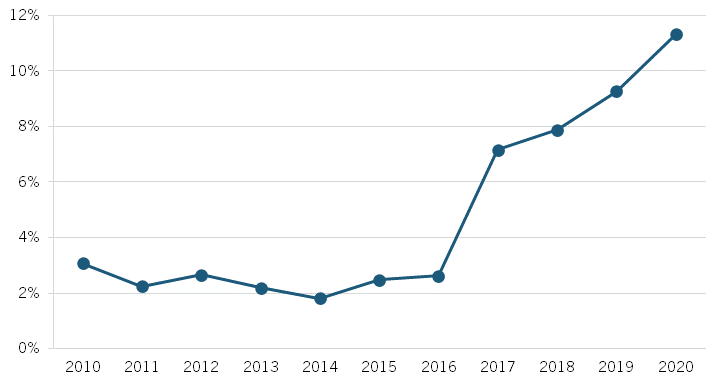
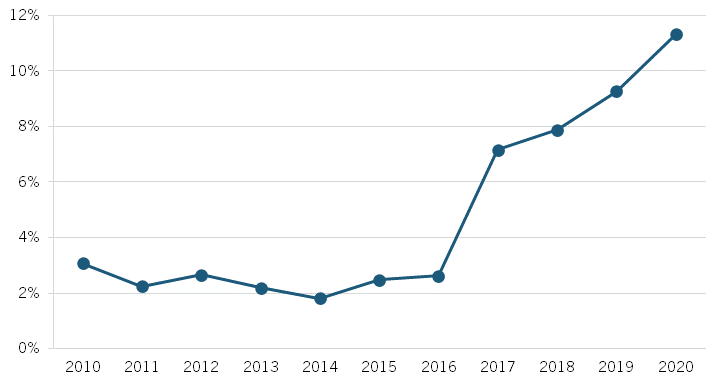
Summary of Innovation Outputs
Innovation outputs are where China made the most progress relative to the United States and where it ended the decade with the largest advantage—and China appears to have shaken off the “fat tech dragon” moniker. By the end of the decade, China published more scientific articles in all fields reviewed except for the geological, atmospheric, and oceanic sciences, but citations data shows that its research is still less influential than the United States’ in all fields except mathematics and statistics. China surpassed the United States in IPFs granted in every field, and it caught up to or surpassed the United States on a per capita basis in telecommunications, pharmaceuticals, biotechnology, and ETs. Incredibly, China received 11.4 times as many ET IPFs in total and more than 2.5 times as many on a per capita basis than did the United States in 2020. Cross-border IP receipts suggest China’s IP is still far less valuable than the United States’. However, China made rapid progress in this regard starting in 2016–2017. Between 2016 and 2020, China’s cross-border IP receipts increased by over 400 percent and its IP receipts as a share of its GDP quadrupled.
INNOVATION OUTCOMES
Production in High-R&D Industries
One important innovation market outcome is increased production in high-R&D industries. The industries included are 1) aircraft manufacturing; 2) computer, electronics, and optical products manufacturing; 3) pharmaceuticals manufacturing; 4) scientific R&D services; and 5) publishing (including software).
Total Production
China’s value added in high-R&D industries amounted to only 34.4 percent of the U.S. level in 2010 (figure 42). China’s progress slowed in the middle of the decade, which corresponds with a slowdown in the broader Chinese economy. But overall, China gained ground steadily, and by 2018, its value added in these industries was 64.1 percent that of the United States. China entered the decade already well ahead of the United States in exports in high-R&D industries, exporting more than twice as much as the United States did in 2010, thanks to its computer, electronics, and optical products exports. Again, China’s progress reversed temporarily in the middle of the decade, but by 2018, China’s exports were almost three times that of U.S. exports in these industries.
Production as a Share of GDP and Exports
However, these figures, while useful, do not account for the size of China’s economy or its overall economic growth during the decade. Considering value added as a share of GDP shows the extent to which an economy specializes in that industry. The same is true for industry exports as a share of total exports, though the specialization is then focused on the goods and services the country produces for the competitive international market.
Rather than considering their absolute values, figure 43 considers each country’s value added and exports in high-R&D industries as shares of their GDPs and total gross exports, respectively. Value added in these industries accounted for 4.1 percent of China’s GDP and 5.1 percent of the United States’ in 2010. By 2018, value added in these industries accounted for 4.7 percent of China’s GDP while the United States’ share was unchanged. Thus, while the United States’ degree of specialization remained essentially unchanged in these industries throughout the decade, China’s increased (to 92.3 percent of the U.S. level).
In 2010, China’s high-R&D exports accounted for 27 percent of gross exports compared with 11.7 percent in the United States. Export specialization in these industries decreased for both countries in the first half of the decade. By 2015, high-R&D exports accounted for 22.6 percent of China’s gross exports and 10.5 percent of the United States’. However, after this point, China reversed course, while export share in the United States continued to decrease. By 2018, these industries accounted for 24.9 percent of China’s exports and 9.8 percent of the United States’.
Figure 43: China’s value added and export shares in R&D-intense industries relative to the United States’95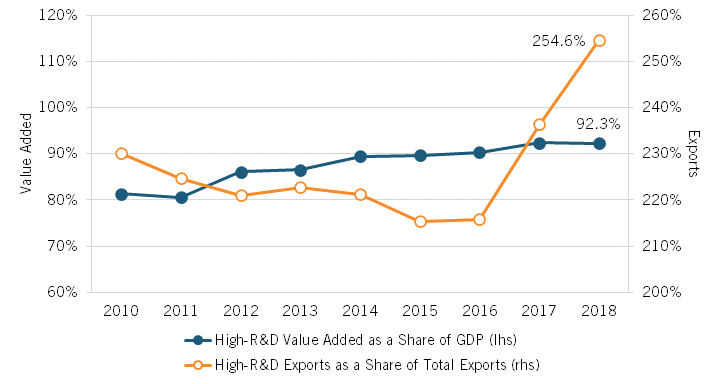
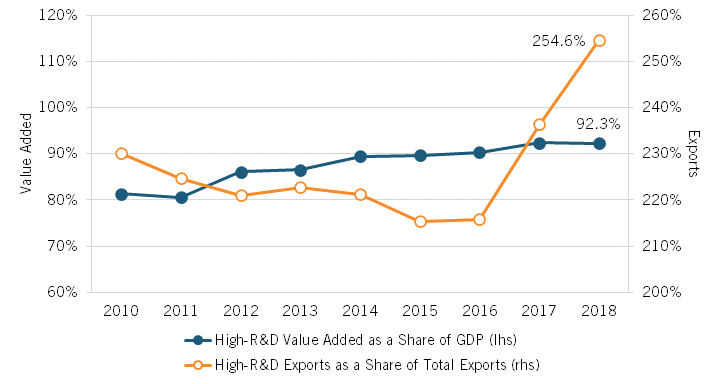
Value Added in Advanced Industries
Total Value Added
Figure 44 and figure 45 carry the value-added analysis further, focusing on seven advanced industries: 1) computer, electronic, and optical products manufacturing; 2) computer programming, consulting, and information services; 3) electrical equipment manufacturing; 4) motor vehicle manufacturing; 5) other transport equipment manufacturing; 6) pharmaceuticals manufacturing; and 7) professional, scientific, and technical activities.
China’s value-added growth in all industries slowed from the first half of the decade to the second, both relative to the United States and in absolute terms. This again corresponds to China’s broader economic slowdown. Nonetheless, China’s value added in each industry relative to the United States was higher in 2018 than in 2010. China’s largest absolute gain was in electrical equipment manufacturing, where its value added increased from 231 percent to 314.2 percent of the U.S. level. China’s largest relative gain was in professional, scientific, and technical activities, which saw its value added relative to the United States double from 2010 to 2018, although this was only from 8.5 to 19.8 percent of the U.S. level. Progress in pharmaceuticals manufacturing was also notable, increasing from 42.2 percent to 73.1 percent of the U.S. level.
China’s fastest individual growth was in the four industries—computer programming, consulting, and information services; other transport equipment manufacturing; pharmaceuticals manufacturing; and professional, scientific, and technical activities—in which China’s value added had not reached U.S. levels. Despite the mid-decade slowdown, these four industries averaged an annual growth rate of 12.2 percent in China from 2010 to 2018, compared with 5 percent for the United States. What’s more, China’s value-added growth was fastest in the two service industries: computer programming, consulting, and information services; and professional, scientific, and technical activities. This suggests that China is transitioning from a manufacturing-based economy to one more dependent on high-value-added services.
Figure 45: China’s value added in advanced industries relative to the United States’ (continued)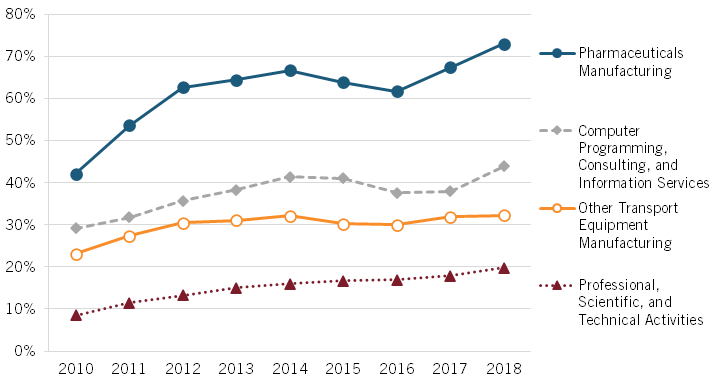
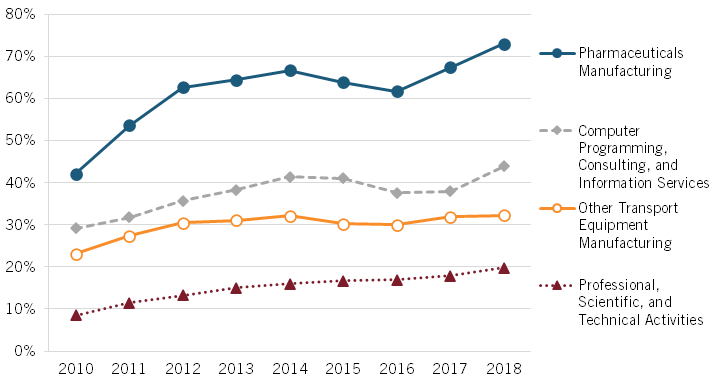
Value Added as a Share of GDP
Figure 46 and figure 47 consider value added as a share of GDP. In all industries except pharmaceuticals manufacturing and professional, scientific, and technical activities, China’s specialization relative to the United States decreased. However, for pharmaceuticals manufacturing, this was because value added in the industry as a share of GDP fell slightly for the United States (rather than China becoming more specialized in the industry). In 2010, value added in the industry accounted for 0.9 percent of GDP for both countries. By 2018, this fell to 0.8 percent for the United States. In contrast, both countries became more specialized in professional, scientific, and technical activities, but China did so at a faster pace. In 2010, value added in the industry accounted for 1.5 percent of China’s GDP and 7.5 percent of the United States’. By 2018, these figures increased to 2.2 percent and 7.7 percent, respectively.
In four of the five industries for which China’s specialization relative to the United States decreased, China’s individual degree of specialization decreased. For example, value added in the electrical equipment manufacturing industry accounted for 1.9 percent of China’s GDP in 2010 but only 1.4 percent in 2018. The exception was computer programming, consulting, and information services. Both countries became more specialized in the industry, but the United States did so at a faster pace. In 2010, value added in the industry accounted for 1.4 percent of China’s GDP and 2 percent of the United States’. By 2018, those numbers increased to 1.8 percent and 2.9 percent, respectively.
Figure 46: China’s value added in advanced industries as a share of GDP relative to the United States’97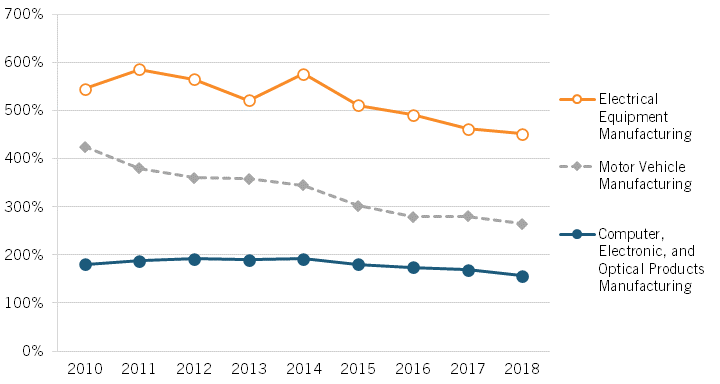
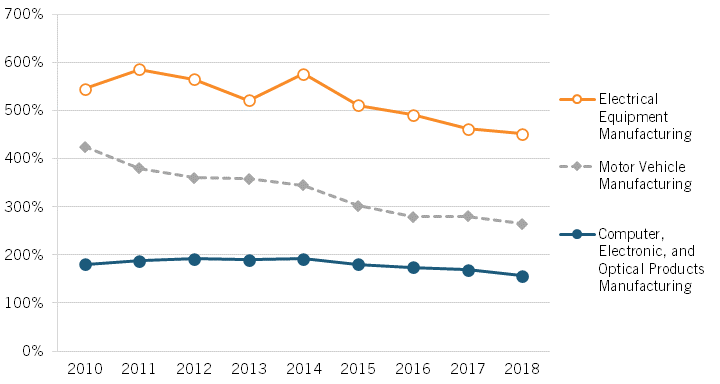
Figure 47: China’s value added in advanced industries as a share of GDP relative to the United States’ (continued)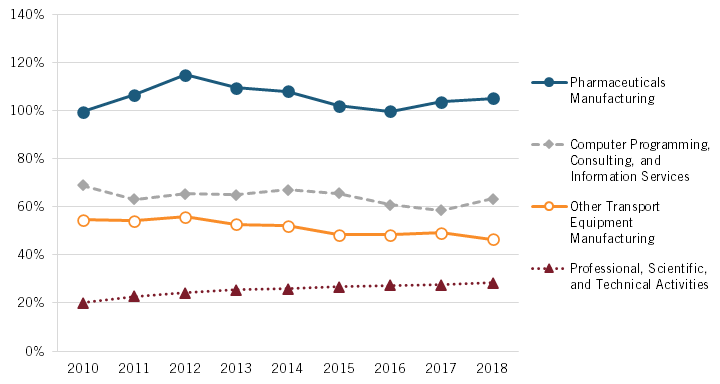
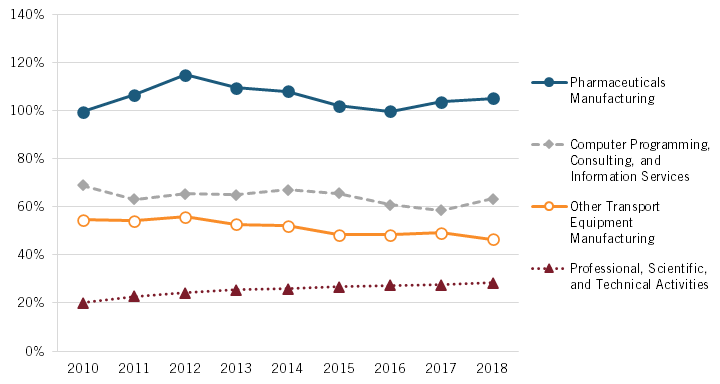
Exports in Advanced Industries
Gross Industry Exports
Figure 48 and figure 49 consider each country’s exports in the advanced industries. China’s exports relative to the United States increased in the following industries: computer, electronic, and optical products manufacturing (from 307.1 percent to 482.3 percent of the U.S. level); computer programming, consulting, and information services (from 89.2 percent to 99.6 percent); electrical equipment manufacturing (from 464.6 percent to 672.4 percent); motor vehicles manufacturing (from 33.8 percent to 43.6 percent); and pharmaceuticals manufacturing (from 27.1 percent to 54.7 percent). China’s exports relative to the United States’ fell for only other transport equipment manufacturing (from 48.6 percent to 37.5 percent of the U.S. level) and professional, technical, and scientific activities (from 27.9 percent to 24.7 percent).
China’s progress relative to the United States was more meager in computer programming, consulting, and information services than in some of the other industries because this is the industry in which each country saw the largest increase in exports. Between 2010 and 2018, China’s exports in the industry grew at an annual rate of 17 percent, while the United States’ grew at an annual rate of 15.4 percent. China also saw significant growth in its pharmaceuticals exports, which increased at an annual rate of 12.8 percent, although the value of these exports fluctuated significantly.
Figure 49: China’s exports in advanced industries relative to the United States’ (continued)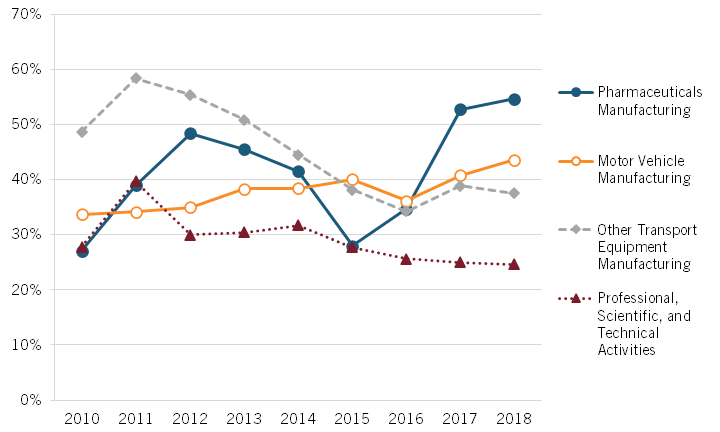
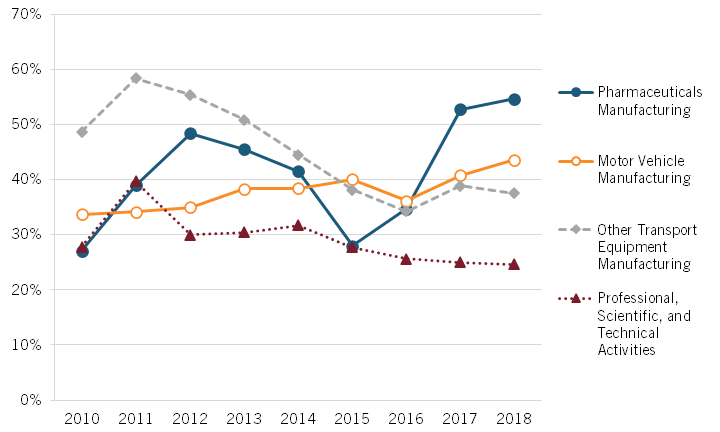
Gross Industry Exports as a Share of Total Gross Exports
No comments:
Post a Comment