ADAM LOWTHER, JAMES PETROSKY, JAMES RAGLAND, ROBYN HUTCHINS
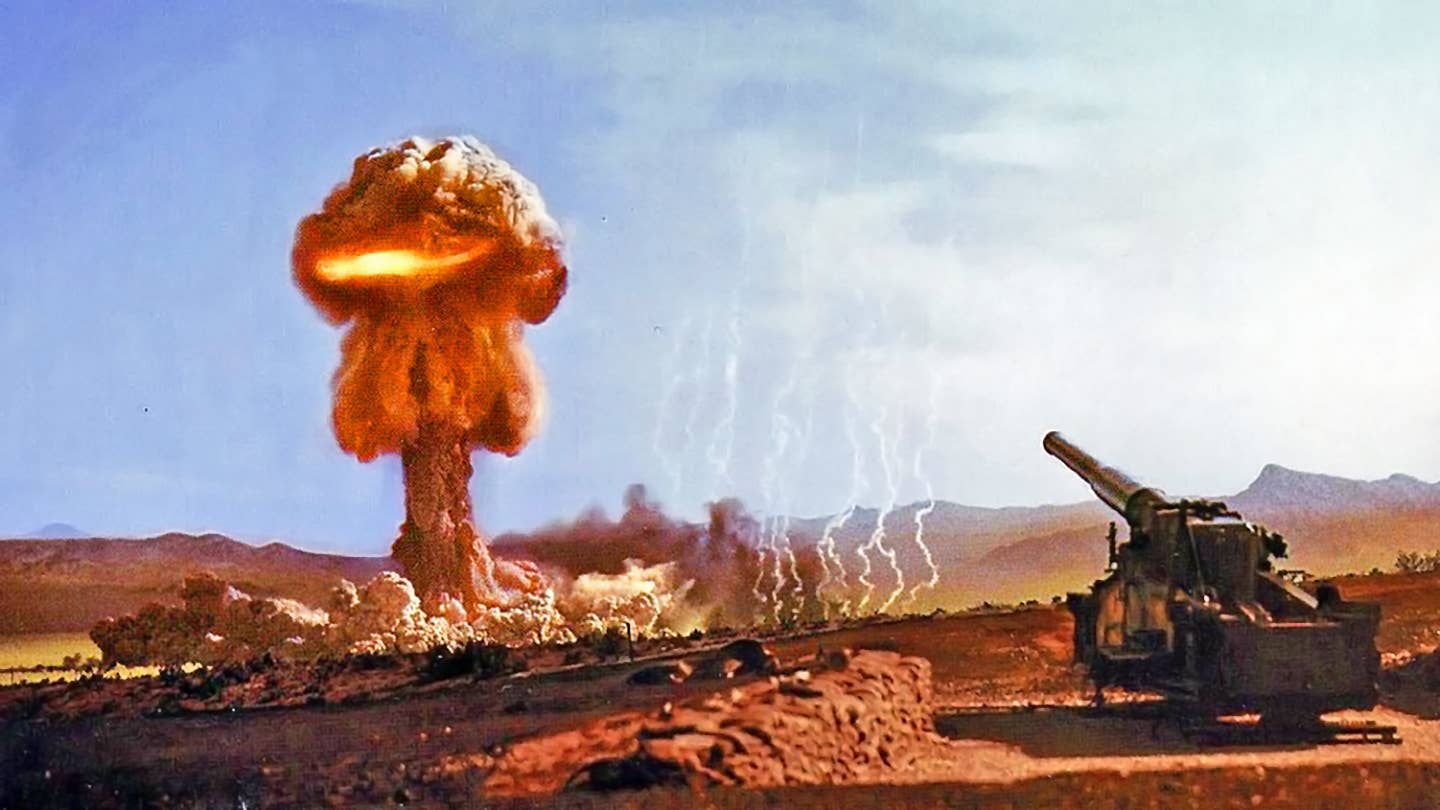
Russian President Vladimir Putin’s repeated nuclear threats against member-states of the North Atlantic Treaty Organization (NATO) for interfering in Russia’s invasion, occupation, and annexation of Ukraine are escalating as the war goes badly for Moscow. His threats are not going unnoticed as the American public shows significant concern amid a renewed interest in the role of nuclear weapons in national security.
For many Americans, any discussion of nuclear weapons use generates images of large-scale exchanges of intercontinental ballistic missiles (ICBM), submarine-launched ballistic missiles (SLBM), and strategic bomber–delivered nuclear cruise missiles. This is, broadly speaking, an image of the United States and Russia engaging in, what the military calls, a full-scale nuclear exchange. Fortunately, this image of nuclear Armageddon is far less likely than a limited use of nuclear weapons on the battlefield in Ukraine. Unfortunately, Russia possesses an estimated 3,000–6,000 low-yield non-strategic nuclear weapons designed for use in just this type of conflict.
Before we go any further, let us specify that the term ‘yield’ is used to compare a nuclear weapon’s energy output by equating it to an equivalent explosive energy of TNT, where a kiloton (kt) is a thousand tons. It is also worth noting that there is no universally accepted yield range for categorizing nuclear weapons. We suggest that a low-yield weapon produces between one and ten kilotons.
For comparison, the nuclear bomb dropped on Hiroshima was about fifteen kilotons. By contrast, the Minuteman III ICBM delivers a nuclear weapon with an estimated yield of 300–475 kilotons. Thus, suggestions that all nuclear weapons are somehow the same belies a lack of understanding of just how powerful some nuclear weapons are, and others are not.

The bomb dropped on Hiroshima code named 'Little Boy." (AtomicArchive.com)
When the 2018 Nuclear Posture Review called for low-yield options like the W76-2 warhead for the Trident D-5 submarine-launched ballistic missile, which has an estimated yield of ten kilotons, it was in an effort to balance against Russian low-yield weapons. It is primarily low-yield weapons that are of most concern for use in Ukraine, not strategic weapons. These weapons produce yields far larger than the GBU-43 Massive Ordnance Air Blast (MOAB) – eleven tons – the largest conventional munition in the American arsenal. Nuclear weapons have a major advantage in their size and weight, and thus are more easily delivered than the GBU-43 to a militarily-relevant target.
By employing low-yield non-strategic nuclear weapons under very specific conditions, nuclear weapons can be employed to dramatically limit the radiation effects associated with nuclear explosions. In this article, we offer a detailed explanation of nuclear weapons effects and the misconceptions many readers have concerning the ways in which it is possible to use non-strategic nuclear weapons while reducing collateral effects associated with nuclear weapons. These attributes are what make them attractive to both Russia and China.

Before moving on it is worth noting that Russia has a nuclear doctrine that calls for escalation to the use of nuclear weapons as a means to de-escalate a conflict that Russian conventional forces are losing. This “escalate to de-escalate” strategy is one that some American analysts question because they view such a doctrine as irrational and hyperbolic. However, Russia's weak conventional military capabilities, compared to the United States and NATO, makes the use of low-yield nuclear weapons one potential way for Russia to stop a potential route of Russian forces in a conflict with NATO. This is not dissimilar from President Eisenhower’s New Look Policy in the 1950s. Ideally, we will never find out if Russia would truly put this doctrine into practice.
Basics of nuclear explosions
To understand how low-yield nuclear explosions produce various effects, an understanding of the basis physics for the energy output is needed. In 1939, German chemist Otto Hahn first observed a neutron bombarding a uranium (U) sample. What Hahn and the rest of the world came to realize is that a neutron, a small particle with a neutral charge, can be absorbed in the nucleus of certain heavy atoms resulting in a new compound nucleus in a highly excited state. To become stable, the highly excited nucleus splits, releasing energy a million times larger than from the atom itself. This is the process commonly referred to as fission.
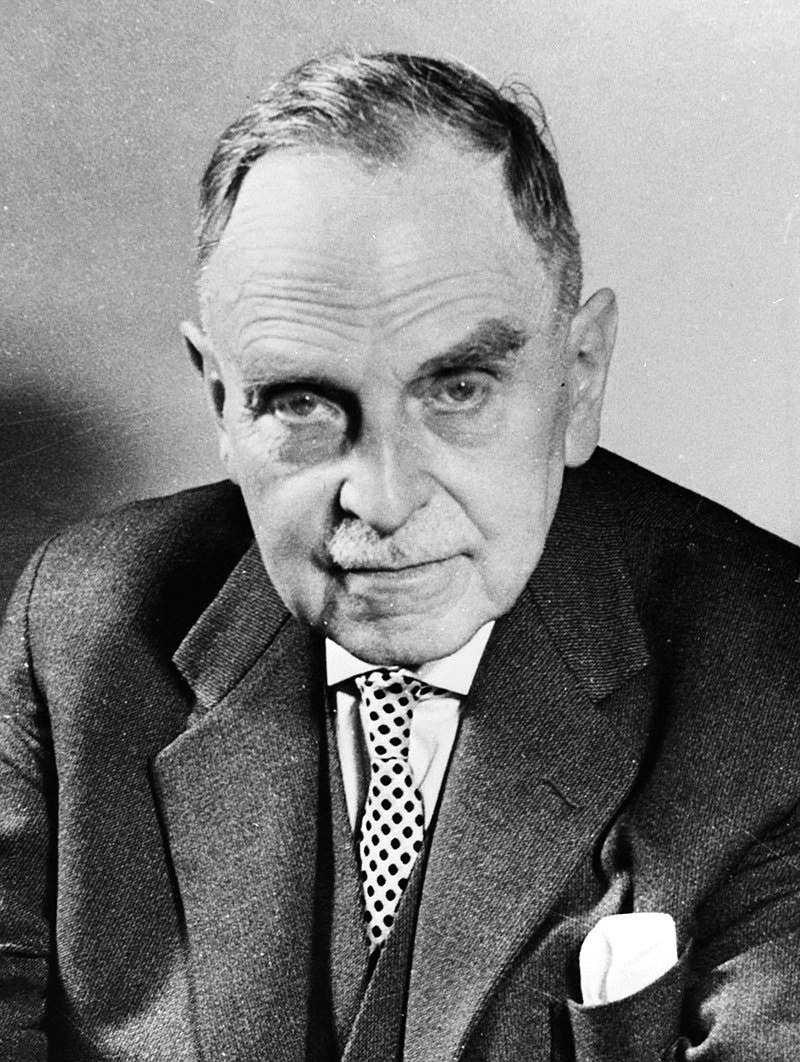
Otto Hahn (Dutch National Archives)
In the fission process, specifically with fissile isotopes of uranium and plutonium, additional neutrons are produced with sufficient energy to cause more fissions. The system can be engineered to create a chain reaction liberating an enormous amount of energy for the mass consumed. This process occurs over a very short time, resulting in a small volume with high energy output. All nuclear weapons rely on this process using a relatively small mass of material (compared to conventional explosives). The engineering required for a nuclear explosion is strongly dependent upon the quality of the fissile material and the ability to maintain the reaction.
Although it may seem contradictory, to achieve a low-yield nuclear explosion, the engineering must be highly advanced to meet the necessary conditions for the nuclear explosion to occur, or the device must be poorly designed and inefficient, wasting valuable fission fuel. Modern American nuclear weapons are small and highly efficient. By contrast, the first nuclear bomb in the American inventory, the Mark-I (15 kilotons), was large and highly inefficient.
Nuclear weapons effects
A nuclear explosion produces several important effects. The primary effects come from blast, thermal radiation, and other radiation. Aside from a few noted scenarios, these mostly scale with yield.
The very short release of energy from a nuclear explosion in the atmosphere results in the formation of a rapidly moving blast wave away from the detonation. It is the primary cause of damage. The blast wave (as calculated from its peak overpressure) is more intense from the same yield than a conventional detonation, making a nuclear detonation militarily significant. The blast wave propagates rapidly and dissipates in a short time. Examples of the effects of the blast can be viewed in nuclear test videos where houses or other buildings are destroyed as the blast wave passes the structure.
In a nuclear explosion, temperatures also reach about 100,000,000 degrees Celsius, resulting in a brilliant fireball. This fireball releases thermal radiation, which damages or destroys nearby objects. Living creatures may suffer burns or death, and flammable materials may catch fire. In the case of Hiroshima, it was the fire that began among the city’s wooden structures that caused more than eighty percent of all damage. Thermal radiation is responsible for about one-third of the damage from a near-surface nuclear explosion. It, too, dissipates rapidly.
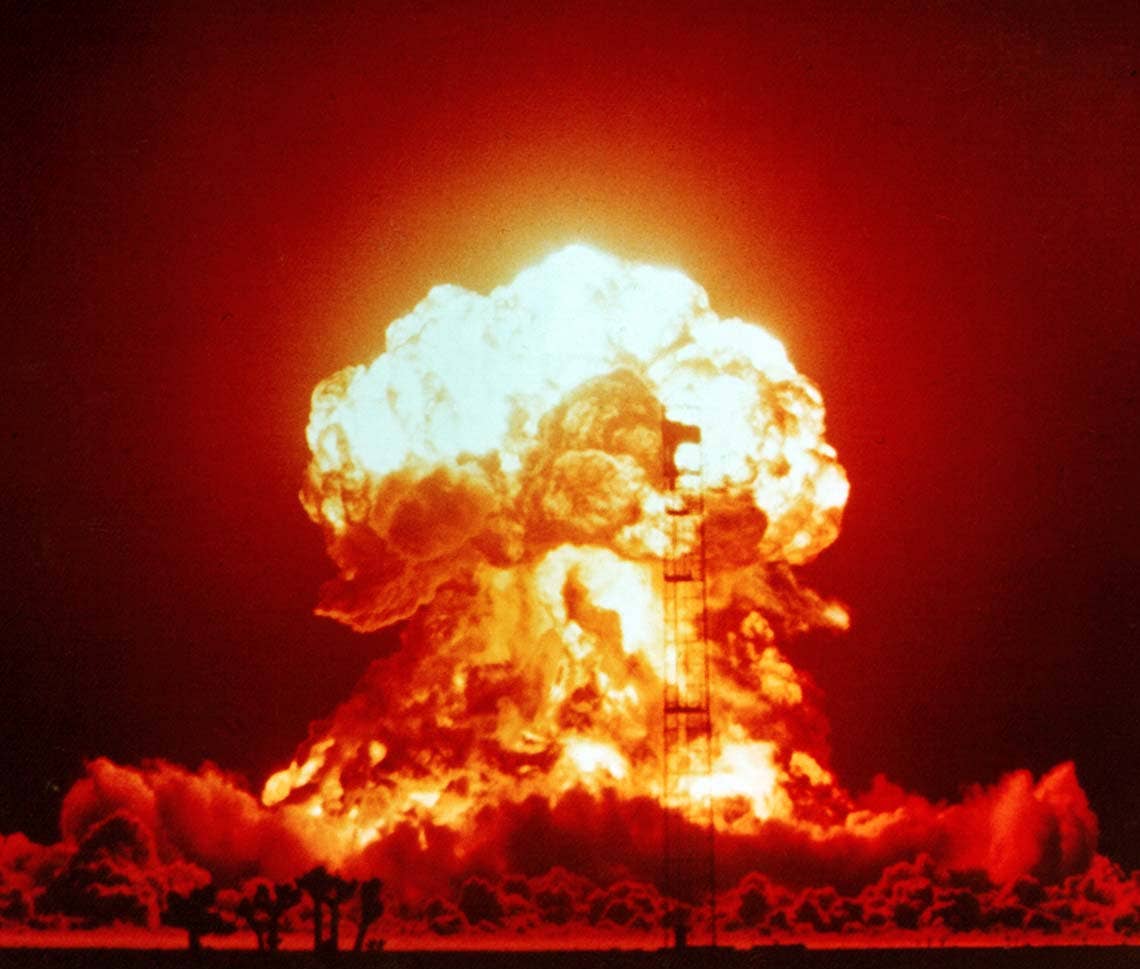
A 23 kiloton tower shot called BADGER, fired on April 18, 1953 at the Nevada Test Site, as part of the Operation Upshot–Knothole nuclear test series. (NNSA)
Unique to nuclear explosions, other radiation can have military effects, even with lower-yield nuclear weapons. Prompt electromagnetic radiation in the form of gamma rays and x-rays and fast neutrons from the detonation are associated with nuclear explosions. Near the earth, these radiation types are stopped at a range determined by absorption in the atmosphere. Therefore, the distance to produce an effect from prompt radiation does not increase appreciably when yield is increased.
Furthermore, excess neutrons from the nuclear explosion or from the decaying split atoms can interact with surrounding material and make it unstable. The unstable atoms release various types of radiation over short to long time periods. For example, if the fireball interacts with the ground, it draws up earth and other debris that become unstable and radioactive. This material is then moved by air currents to other locations and falls to the ground, depositing the radiation referred to as fallout. If, however, the fireball does not touch the ground, then only the bomb materials not consumed in the fireball (missile or bomb material) are made radioactive, substantially reducing the amount of radioactive material.
One result of the more than one thousand nuclear tests conducted by the United States is that American scientists developed effective calculations for understanding nuclear weapons effects. The MIT nuclear weapons education project and NUKEMAP apply the basic calculations (with their own modifications) found in Samuel Glasstone and Philip J. Dolan’s The Effects of Nuclear Weapons, which is perhaps the best initial resource for understanding nuclear weapons effects.
Evaluating low-yield nuclear weapons effects
The Defense Threat Reduction Agency (DTRA) maintains high-fidelity nuclear weapons effects software that provides detailed information relating to effects and the variables that shape them. Unfortunately, an unclassified version of their software is not available. Thus, calculations for this article were derived using the unclassified Los Alamos Simple Effects Calculator.
Many variables affect the outcome of a nuclear detonation. Atmospheric conditions like rain, clouds, wind, and humidity change many aspects of the overall effect in radiation propagation and fallout. Topography also plays a role in a blast as, for example, mountains reflect blast waves and cityscapes channel winds. Since most nuclear tests were performed in open areas (deserts and oceans), much national effort was applied to understanding these other effects. However, since they are all based upon specific scenarios, public weapons effects calculators, such as the one used in this article, are limited in their ability to provide fidelity for all effects.
In previous work, we examined the use of a ten kiloton “fallout free” nuclear air burst that could be tailored to serve as a demonstration strike on a military target. The primary weapons effects – blast (overpressure), thermal radiation, and prompt radiation – were calculated from ground zero. Table 1 offers expected values for each of the three main effects of a ten-kiloton detonation, providing the distance to meet a relevant damage threshold.
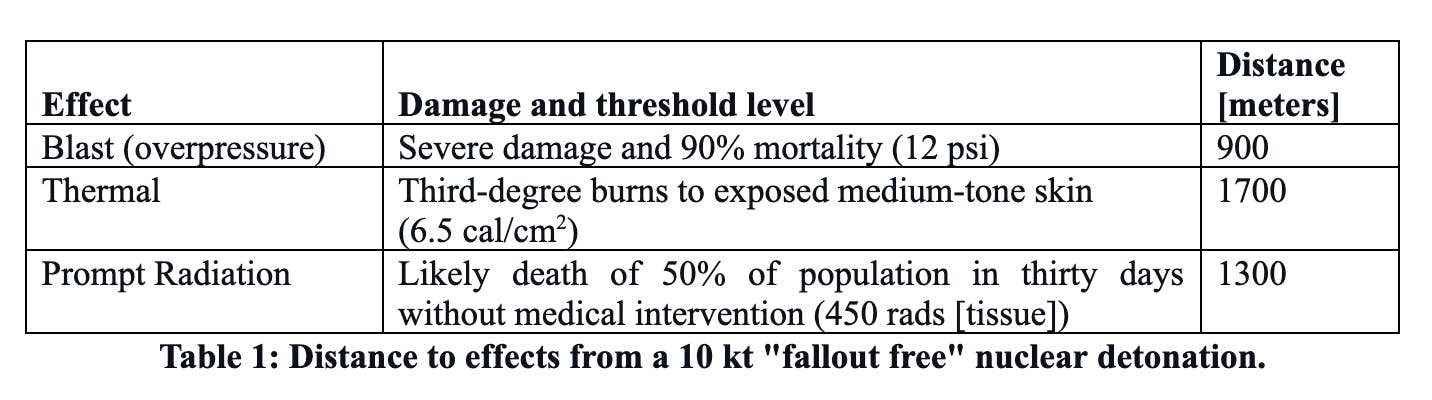
Table 1 illustrates that the major damaging effects from overpressure, thermal radiation, and prompt radiation from a ten-kiloton low-altitude detonation are not far-reaching. As a reminder, the prompt radiation is instantaneous and not long-lasting like fallout. This runs counter to the impression of many who believe any nuclear detonation turns the surrounding landscape into an irradiated wasteland. China and Russia understand this as well and see the military utility of low-yield nuclear weapons which, because of their smaller yields, would have even smaller damage footprints.
Below we provide further details about the effects of low-yield nuclear detonations. Three nuclear weapon detonations of one-, ten-, and twenty-five-kiloton yields are evaluated to determine the extent of damage at yields that are all possible with Russian non-strategic nuclear weapons. The twenty-five-kiloton yield weapon is outside our definition for a low-yield weapon but offers a useful size for sake of comparison.
In addition to providing effect calculations for three yields, we calculate for a ground detonation and air burst as these produce substantially different effects. For a ground burst, the fireball draws up considerable ground debris and reflects the blast wave. These result in differences in fallout and blast wave energy.

For air bursts, we calculate effects at a height of burst (HOB) that is both “fallout free” and optimizes overpressure to 12 psi. This is enough overpressure to cause severe damage to concrete structures, meaning the structure can no longer be used for its designed purpose. It also has a combined acute effective mortality rate of approximately ninety percent in the blast zone.
We also determined the distance from ground zero out to which an individual would receive an absorbed dose of approximately 450 rad [Ti] (radiation-absorbed dose to tissue). We use 450 rad [Ti] because this is the amount of radiation required to cause a lethal dose in fifty percent of the exposed population within thirty days absent medical attention. To reach this dose, a soldier, for example, would need to stand on the surface completely unprotected within the affected blast zone. In the case of a soldier sheltering inside a building, trench, or vehicle, for example, the dose would be reduced.
We also calculate the estimated hourly dose from residual radiation received by an individual within 1,000 meters of ground zero (GZ) for 24, 48, and 96 hours after detonation. This provides important data because personnel may need to move through an area where a nuclear weapon was detonated. Thus, residual radiation plays a role in increasing or decreasing effects from a nuclear detonation. In Table 2, we use Roentgen equivalent man (rem) to describe radiation dosage. The unit of rem accounts for the radiation type and its effect on a human. In general, 0.87 rem equals 1 rad.

Several important general observations are visible from Table 2. First, even though the twenty-five-kiloton detonation is twenty-five times higher in energy than the one-kiloton detonation, the circumference of the 450-rad threshold is only 1.3 times further out for the twenty-five-kiloton detonation than the one-kiloton detonation. This illustrates that it is not possible to assume that yield and weapons effects maintain a perfect relationship as yield increases. Second, the residual radiation is less than half its original value within 48 hours for all detonations. Residual radiation declines rapidly. Third, even for a larger detonation, like twenty-five kilotons, the circumference from ground zero of deadly weapon effects only goes out to a little more than one kilometer in the worst case. For smaller yields, devastation exists in a much smaller area.
For the one-kiloton detonation, the overpressure created in a ground burst—sufficient to collapse concrete structures—extends out 285 meters from ground zero. In the case of an air burst the distance is 362 meters. While the air burst is more effective in creating overpressure, the residual radiation of an air burst is significantly less than the ground burst since debris does not interact with the neutron radiation. It is worth pointing out that a one-kiloton low-yield nuclear weapon is roughly 100 times more powerful than a GBU-43 MOAB (11 tons of TNT), which makes it an attractive weapon for Putin, particularly as an air-burst weapon against troops in the open.
For a ten-kiloton detonation, an air burst is more effective in creating the overpressure needed to collapse concrete structures. But, as our calculations suggest, this is true up to 779 meters from ground zero. For a ground burst, effective range is decreased by about 150 meters. An air burst also produces about five percent of the residual radiation generated by a ground burst, which is important if an area is occupied after detonation or if reduced long-term radiation effects are desired. This point is underscored by the very low residual radiation present at 24, 48, and 96 hours after an air bust.

The twenty-five-kiloton detonation is interesting because the primary military effects of blast and prompt radiation do not scale linearly. For example, the twenty-five-kiloton yield is 2.5 times larger than the ten-kiloton detonation, but for the surface bursts, the twenty-five-kiloton detonation’s 450-rad range is only 1.1 times larger than the ten kiloton’s range. However, the residual radiation at 24 hours is 2.5 times larger. Again, the twenty-five-kiloton weapon is not as efficient at producing the needed military effects and is more effective at producing potentially unwanted effects.
A final point worth noting is that much of the analysis leading to these estimates was designed to assist the U.S. Army in understanding nuclear weapons effects on American soldiers fighting on a battlefield where the Soviet Union could employ nuclear weapons. For very practical reasons, the Army needed to know how to continue fighting. Thus, the calculations presented here are related to the fighting capacity of soldiers exposed to radiation on a battlefield.
Conclusions
Our objective in writing this article was to illustrate that low-yield weapons do not cause the widespread devastation and radiological disaster that is considered common knowledge. As practitioners with decades of experience inside the nuclear enterprise, we often take for granted that many Americans do not have the same education, training, experience, and access to specialized tools and knowledge that enables many within our community to think through these issues with great fidelity.
It is also important to understand that presenting a complex topic like nuclear weapons effects in a relatively brief article, written for interested laymen, requires a reduction of detail so that concepts are easily digested and understood. Inasmuch as is possible, we present data that is supportable within broad assumptions. Our analysis reduces specific detail, but it supports the conclusion that for actors like Russia and China, lower-yield nuclear weapons have military value tailored for use on the battlefield and for demonstration strikes that limit or eliminate long-term radiological effects. Still, there are many other serious considerations beyond these factors pertaining to their use, especially in terms of risk of rapid escalation and the precedent their use would set, even on a limited scale.
To underscore this point, all three of the low-altitude air bursts, for which we calculated effects, produced important military effects and almost eliminate residual radiation. Even in the case of a twenty-five-kiloton air burst, at 613 meters above ground zero, 96 hours after detonation, the hourly residual radiation dose rate for an unprotected person within 1,000 meters of ground zero is 4 rems – a dose that is within the Department of Energy five rem yearly limit for occupational radiation workers. If the burst were not optimized for overpressure and the destruction of concrete structures, a detonation even a few hundred meters further above ground zero, like at Hiroshima, would produce almost no residual radiation at all.
China and Russia know and understand nuclear weapon effects well and are developing the very weapons needed to destroy targets without creating long-term radiological disasters. They are filling the gaps in their conventional forces with much lower yield options that are imminently usable. Thinking through these possible situations is certainly in the nation’s interest as the United States continues to support Ukraine’s defense.
No comments:
Post a Comment